Five Practical Uses for “Spooky” Quantum Mechanics
Fifty years after Bell’s Theorem, tools that harness the weird properties of quantum mechanics are at work all around you
/https://tf-cmsv2-smithsonianmag-media.s3.amazonaws.com/filer/9d/02/9d02fd07-b2b0-4ae4-afbb-65bef25d86a0/42-46209404.jpg)
Quantum mechanics is weird. The theory, which describes the workings of tiny particles and forces, notoriously made Albert Einstein so uneasy that in 1935 he and his colleagues claimed that it must be incomplete—it was too “spooky” to be real.
The trouble is that quantum physics seems to defy the common-sense notions of causality, locality and realism. For example, you know that the moon exists even when you’re not looking at it—that's realism. Causality tells us that if you flick a light switch, the bulb will illuminate. And thanks to a hard limit on the speed of light, if you flick a switch now, the related effect could not occur instantly a million light-years away according to locality. However, these principles break down in the quantum realm. Perhaps the most famous example is quantum entanglement, which says that particles on opposite sides of the universe can be intrinsically linked so that they share information instantly—an idea that made Einstein scoff.
But in 1964, physicist John Stewart Bell proved that quantum physics was in fact a complete and workable theory. His results, now called Bell’s Theorem, effectively proved that quantum properties like entanglement are as real as the moon, and today the bizarre behaviors of quantum systems are being harnessed for use in a variety of real-world applications. Here are five of the most intriguing:
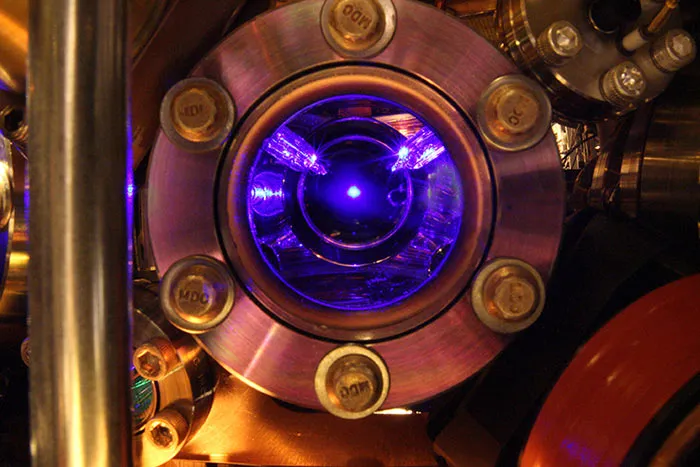
Ultra-Precise Clocks
Reliable timekeeping is about more than just your morning alarm. Clocks synchronize our technological world, keeping things like stock markets and GPS systems in line. Standard clocks use the regular oscillations of physical objects like pendulums or quartz crystals to produce their ‘ticks’ and ‘tocks’. Today, the most precise clocks in the world, atomic clocks, are able to use principles of quantum theory to measure time. They monitor the specific radiation frequency needed to make electrons jump between energy levels. The quantum-logic clock at the U.S. National Institute of Standards and Technology (NIST) in Colorado only loses or gains a second every 3.7 billion years. And the NIST strontium clock, unveiled earlier this year, will be that accurate for 5 billion years—longer than the current age of the Earth. Such super-sensitive atomic clocks help with GPS navigation, telecommunications and surveying.
The precision of atomic clocks relies partially on the number of atoms used. Kept in a vacuum chamber, each atom independently measures time and keeps an eye on the random local differences between itself and its neighbors. If scientists cram 100 times more atoms into an atomic clock, it becomes 10 times more precise—but there is a limit on how many atoms you can squeeze in. Researchers’ next big goal is to successfully use entanglement to enhance precision. Entangled atoms would not be preoccupied with local differences and would instead solely measure the passage of time, effectively bringing them together as a single pendulum. That means adding 100 times more atoms into an entangled clock would make it 100 times more precise. Entangled clocks could even be linked to form a worldwide network that would measure time independent of location.
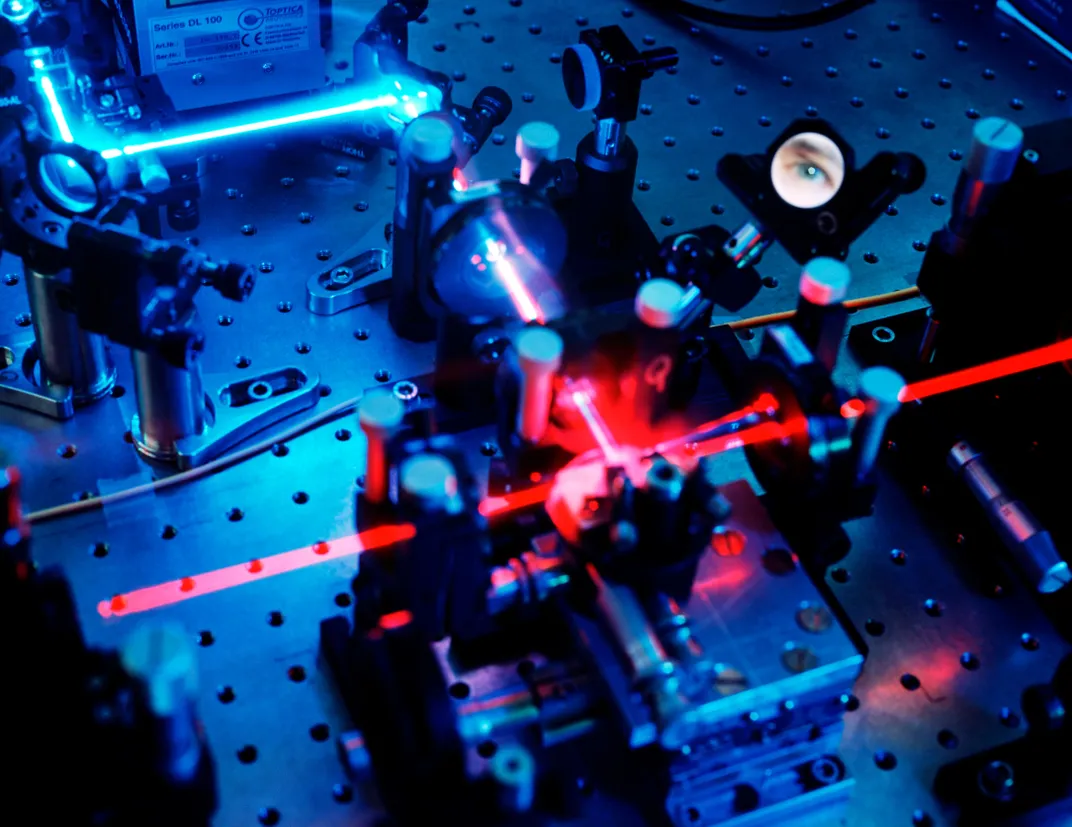
Uncrackable Codes
Traditional cryptography works using keys: A sender uses one key to encode information, and a recipient uses another to decode the message. However, it’s difficult to remove the risk of an eavesdropper, and keys can be compromised. This can be fixed using potentially unbreakable quantum key distribution (QKD). In QKD, information about the key is sent via photons that have been randomly polarized. This restricts the photon so that it vibrates in only one plane—for example, up and down, or left to right. The recipient can use polarized filters to decipher the key and then use a chosen algorithm to securely encrypt a message. The secret data still gets sent over normal communication channels, but no one can decode the message unless they have the exact quantum key. That's tricky, because quantum rules dictate that "reading" the polarized photons will always change their states, and any attempt at eavesdropping will alert the communicators to a security breach.
Today companies such as BBN Technologies, Toshiba and ID Quantique use QKD to design ultra-secure networks. In 2007 Switzerland tried out an ID Quantique product to provide a tamper-proof voting system during an election. And the first bank transfer using entangled QKD went ahead in Austria in 2004. This system promises to be highly secure, because if the photons are entangled, any changes to their quantum states made by interlopers would be immediately apparent to anyone monitoring the key-bearing particles. But this system doesn't yet work over large distances. So far, entangled photons have been transmitted over a maximum distance of about 88 miles.
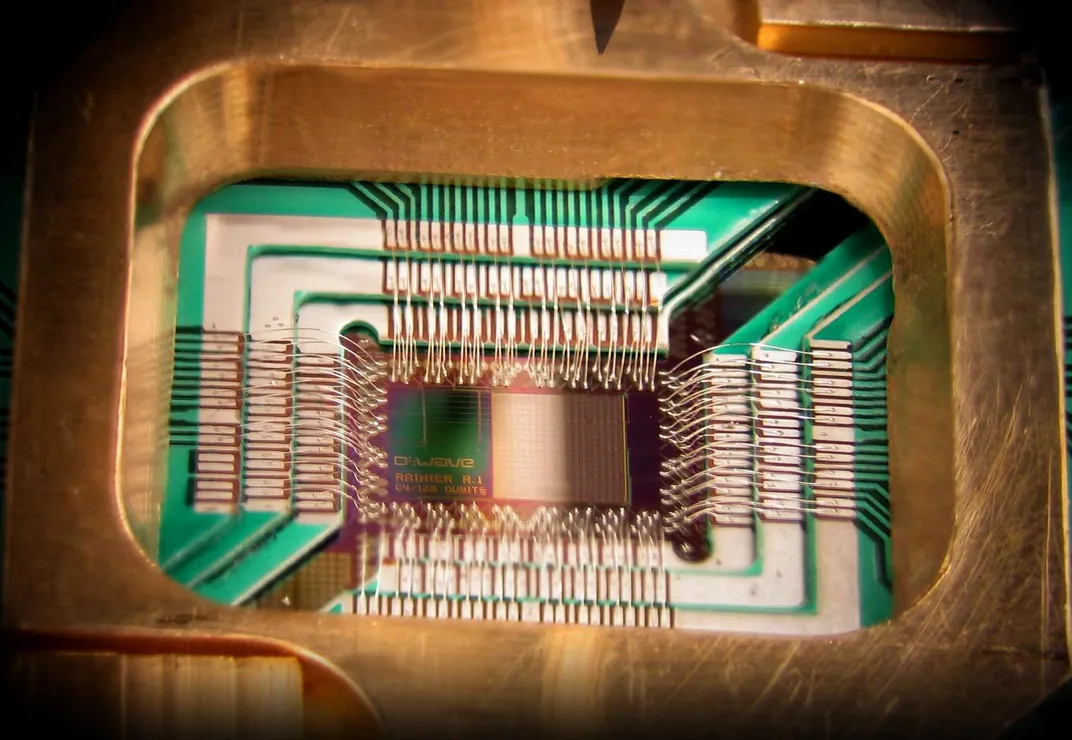
Super-Powerful Computers
A standard computer encodes information as a string of binary digits, or bits. Quantum computers supercharge processing power because they use quantum bits, or qubits, which exist in a superposition of states—until they are measured, qubits can be both "1" and "0" at the same time.
This field is still in development, but there have been steps in the right direction. In 2011, D-Wave Systems revealed the D-Wave One, a 128-qubit processor, followed a year later by the 512-qubit D-Wave Two. The company says these are the world's first commercially available quantum computers. However, this claim has been met with skepticism, in part because it’s still unclear whether D-Wave’s qubits are entangled. Studies released in May found evidence of entanglement but only in a small subset of the computer’s qubits. There's also uncertainty over whether the chips display any reliable quantum speedup. Still, NASA and Google have teamed up to form the Quantum Artificial Intelligence Lab based on a D-Wave Two. And scientists at the University of Bristol last year hooked up one of their traditional quantum chips to the Internet so anyone with a web browser can learn quantum coding.
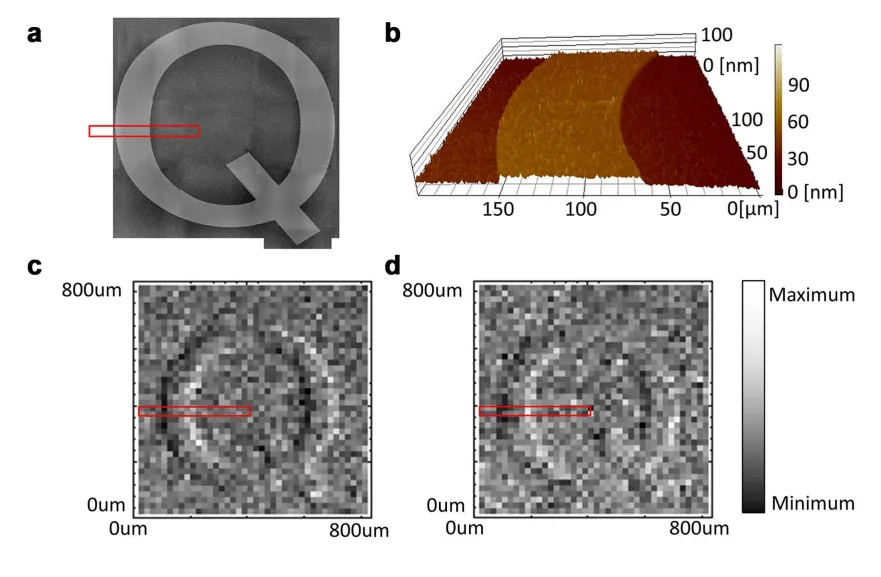
Improved Microscopes
In February a team of researchers at Japan’s Hokkaido University developed the world’s first entanglement-enhanced microscope, using a technique known as differential interference contrast microscopy. This type of microscope fires two beams of photons at a substance and measures the interference pattern created by the reflected beams—the pattern changes depending on whether they hit a flat or uneven surface. Using entangled photons greatly increases the amount of information the microscope can gather, as measuring one entangled photon gives information about its partner.
The Hokkaido team managed to image an engraved "Q" that stood just 17 nanometers above the background with unprecedented sharpness. Similar techniques could be used to improve the resolution of astronomy tools called interferometers, which superimpose different waves of light to better analyze their properties. Interferometers are used in the hunt for extrasolar planets, to probe nearby stars and to search for ripples in spacetime called gravitational waves.
/https://tf-cmsv2-smithsonianmag-media.s3.amazonaws.com/filer/d4/c0/d4c0a398-bb8c-43d0-bc75-29b190ce29b3/42-22198909.jpg)
Biological Compasses
Humans aren't the only ones making use of quantum mechanics. One leading theory suggests that birds like the European robin use the spooky action to keep on track when they migrate. The method involves a light-sensitive protein called cryptochrome, which may contain entangled electrons. As photons enter the eye, they hit the cryptochrome molecules and can deliver enough energy to break them apart, forming two reactive molecules, or radicals, with unpaired but still entangled electrons. The magnetic field surrounding the bird influences how long these cryptochrome radicals last. Cells in the bird’s retina are thought to be very sensitive to the presence of the entangled radicals, allowing the animals to effectively ‘see’ a magnetic map based on the molecules.
This process isn't full understood, though, and there is another option: Birds' magnetic sensitivity could be due to small crystals of magnetic minerals in their beaks. Still, if entanglement really is at play, experiments suggest that the delicate state must last much longer in a bird’s eye than in even the best artificial systems. The magnetic compass could also be applicable to certain lizards, crustaceans, insects and even some mammals. For instance, a form of cryptochrome used for magnetic navigation in flies has also been found in the human eye, although it’s unclear if it is or once was useful for a similar purpose.