In science, inspiration can come from anywhere.
A researcher might wonder about the thrust produced by butterfly wings as they flit through a meadow. Maybe watching squirrels navigate campus trees like parkour artists sparks curiosity about what goes through their furry little minds as they decide to jump from branch to branch.
Others, of course, get their spark from less likely sources: analyzing shark intestines or studying how microscopic water bears walk.
These scenarios and more inspired scientists to take a closer look at the natural world this year, but their influence won’t stop there. Discoveries in nature often inspire new designs for water filtration, solar panels, building materials and lots and lots of robots.
Here are ten findings from 2021 that could one day lead to new inventions.
Shark Intestines Function Like Tesla Valves
/https://tf-cmsv2-smithsonianmag-media.s3.amazonaws.com/filer_public/ee/07/ee077f30-06f7-4bf7-96b9-27b5c86a336c/20-july_shark-intestines-tesla-valve.jpeg)
In 1920, Serbian-American inventor Nikola Tesla designed a device without any moving parts that allows fluid to move in one direction while preventing backflow. These valvular conduits, as Tesla himself called them, are made of a series of fixed, tear-shaped loops that allow fluid to easily travel through the device, but slow and block any returning liquids. He made the valves as part of a new steam engine he was working on, but filed for bankruptcy several months later, leaving the patent forgotten for decades.
Intestines serve a similar purpose: absorb nutrients from food and flush out waste, which certainly shouldn’t go out the way it came in.
In the animal kingdom, intestines come in all shapes and sizes. Most creatures—including humans—have tubular intestines that require muscle contractions to push food through. But shark intestines slowly move digested meals through spring-shaped spirals or nested funnels, depending on the species, to eke out every last calorie and get rid of the rest.
This year, researchers found that these spiraled organs also resemble the design and function of Tesla valves. The twists, turns and funnels ease fluids forward, which could be an energy-saving form of digestion.
“Sharks have all these different little tweaks to the Tesla valve design that could be making them more efficient,” study author Samantha Leigh, an animal physiologist at California State University, Dominguez Hills, told New Scientist in July.
Studying these natural wonders of engineering could improve wastewater filtration systems for removing microplastics. “We plan on doing work this upcoming year to determine how effective the different morphologies are at filtering out various size particles,” Leigh tells Smithsonian via email. “Depending on the results, we might be able to ‘tweak’ the spiral intestine design to make filters that are as effective as possible at filtering out microplastics from storm [or] wastewater.”
Squirrels Can Teach Robots a Lesson About Agility and Recovery
When navigating treetops, squirrels will risk a long-distance leap if there’s a sturdy branch to land on. But even if their next stop is flimsy, a squirrel’s expert-level recovery skills—possibly developed from past mistakes—almost always help them stick the landing.
These two lessons from our fuzzy friends could be useful in designing how agile robots analyze a target to jump toward and how they recoup missed marks, according to research published in August.
“Like squirrels, the next generation of fast, agile legged robots could perceive opportunities to exploit specific aspects of the world around them that directly complement their own capabilities,” study author Nathaniel Hunt, a biomechanics engineer at the University of Nebraska, says via email. “Sensors and perception may be designed to directly identify only the critical information that supports movement decisions and control while ignoring a huge amount of irrelevant information.”
To learn more about how squirrels successfully navigate from branch to branch, scientists put wild fox squirrels to the test on an obstacle course in a eucalyptus grove on the University of California, Berkeley campus. The team analyzed how the squirrels altered their jumps depending on branch flexibility—timing their launch a bit earlier—and how they geared up for distance and height variation—twisting mid-air to find something to bounce off of to give them a boost. After going through a tricky jump a few times, they eventually nailed the stunt with ease.
Engineers designing robots for “inspection and maintenance, working in the home, and even exploration” can learn a few things from these bushy-tailed rodents, explains Hunt.
“As robots move out of laboratories and start to do work in the real world, somehow they have to deal with the complexity of interacting with different surfaces and objects, various materials that are hard or soft, smooth or rough, and they must react appropriately when they bump into something, lose traction or some other unexpected thing happens,” he says.
Scavenger Beetles Walk Upside-Down Beneath the Water’s Surface
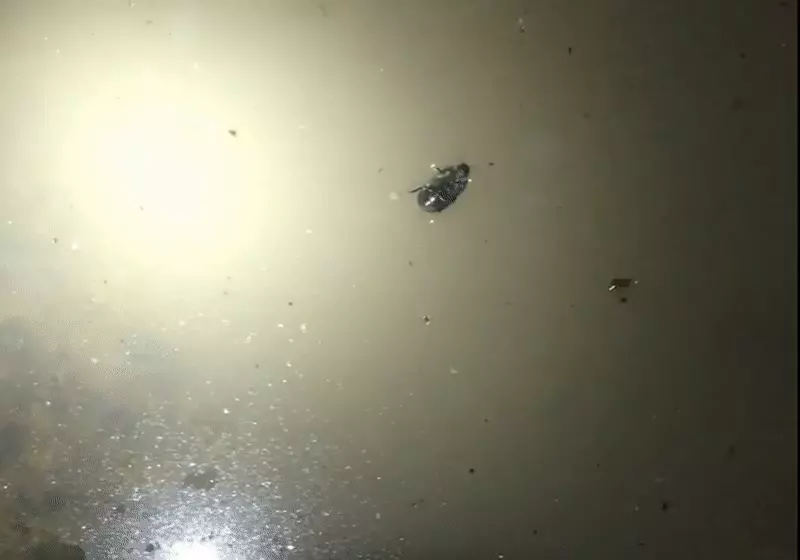
Thanks to surface tension, spiders, ants, snails and lizards can all walk on water, but water scavenger beetles perform a trickier feat. These bugs flip upside-down and scuttle along just beneath the water’s surface—as if they’re clinging to the underside of a glass table.
"The moment I realized the beetle was moving across the underside of the water's surface, I knew I had found something truly bizarre," says behavioral biologist John Gould, per Live Science.
In the first study to analyze this skill in depth, researchers filmed the insects’ inverted crawling technique. These beetles are known to trap air bubbles with their leg hairs to keep them oxygenated, but video footage shows this behavior may also keep them afloat and pinned to the surface. The bubble likely gives the bug enough support to put some pressure on the water-air boundary without breaking through. The beetle’s footsteps even push up “tiny hills” of water along the surface as they go, Gould tells Science News.
Scientists still have plenty of questions about the physiology of the beetle itself, like how its foot anatomy affects this skill or whether different parts of its body are water resistant. Studying the scavenger beetle’s talents could inspire tiny, upside-down surfing aquatic robots, Gould says via email.
Some water-skimming micro-robots already exist, and engineers are also building bots that can move between terrestrial and aquatic environments. However, current models require “multiple locomotion styles or inhibit the locomotion in one environment for the sake of the other,” Gould says. A beetle-bot could eliminate the need for hybrid models because the device would walk on land the same way it can walk underwater. “We believe that this could further advance robot locomotive capabilities for military or search and rescue purposes,” he adds.
Not only could the beetle in the study move beneath the surface, it could also remain motionless in one spot. A beetle-inspired aquatic adhesive or structural design could also be a solution for keeping objects at the surface of the water to measure vibrations, take temperature readings or collect other data.
“It could be possible to synthesize materials which can exploit this feat and remain passively at the water’s surface without the expenditure of energy,” Gould says. “Devices made of these materials could remain at the surface of the water while remaining entirely submerged and without piercing through or disrupting the water’s surface, unlike other devices which require a flotation device that sits above the water.”
A Cancer-Defying Gecko and His 900 Babies Could Inspire New Melanoma Treatments
/https://tf-cmsv2-smithsonianmag-media.s3.amazonaws.com/filer_public/d4/40/d440e127-bebd-438c-9aff-7a8811bb59f1/superlemonfrost-1430px.jpeg)
At an auction in 2015, reptile breeder Steve Sykes purchased a rare pair of “lemon frost” geckos for $10,000. But when he started breeding the male gecko, Mr. Frosty, he noticed many of the offspring had small, white tumors growing on their skin. Nearly 80 percent of lemon frost geckos—a type of genetic morph bred for their sunny color—will develop this skin cancer that arises from pigment-producing cells called iridophores.
University of California, Los Angeles geneticist Longhua Guo happened to reach out to Sykes to study gecko morphs and decided to look into the genetic mystery of the lemon frost geckos. Guo and his team found a gene called SPINT1, which is linked to skin cancer in humans, is also responsible for both the geckos’ golden glow and their tumors. Studying SPINT1 further could help scientists better understand how certain cancers develop in humans—and perhaps lead to new ways to treat the illness.
Guo would like to “try culturing the cancerous iridophores to develop a new cell line for investigating tumor pathways as well as potential treatments,” per The Scientist.
Speaking with Scientific American in June, Lara Urban, a genome researcher at the University of Otago in New Zealand who was not involved in the study, says studying how the gene is expressed in geckos that never develop cancer could be one path for future study.
“I do think it will have an impact on cancer research, in that we understand the conservedness of this [SPINT1 genetic] pathway a little bit better now,” Urban says. “It will also be a potential new model organism for studying the development of skin cancer and contributing to actual therapeutic development.”
Scientists Watch Butterfly Wings Grow Scales for the First Time
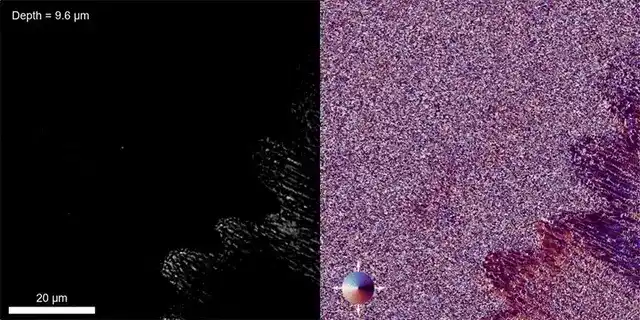
For the first time ever, MIT researchers peered into a chrysalis while the colorful scales on a butterfly wing formed—and captured continuous images of the process, according to a study published this month in the journal PNAS. Studying this process could one day inspire new multi-functional materials in vibrant colors that also provide temperature control and water resistance, says study author Mathias Kolle, a mechanical engineer at MIT.
“We believe that being able to see and understand the processes and control levers at the core of the butterflies’ ability to tailor material structure and performance will help us to sustainably translate them into strategies for forming the next generation of advanced functional materials,” he tells Smithsonian via email.
First, the team raised painted lady (Vanessa cardui) caterpillars until they entered metamorphosis and developed a chrysalis. Then, they carefully removed part of the outer casing and placed a transparent coverslip over the opening using a bioadhesive. With a window into the transformation, the team used speckle-correlation reflection phase microscopy to get a closer look. Instead of using a wide-beam light that could be phototoxic to the delicate wing, speckle-correlation reflection phase microscopy peppers small points of light over specific locations, creating a field of illumination like fireflies in a meadow at dusk.
Within days, cells lined up to form rows of scales in an alternating pattern above and beneath the wing, like shingles on a roof. Each scale then developed nanometer-high grooves. Researchers suspect these ridges help the butterflies shed water like microscopic rain gutters as well as collect heat to keep them dry.
“We think this work could lead to new inventions because butterfly scales are a great biological example of a material solution with multiple coveted functions, all enabled by the same structure,” Kolle says. “These functions include control over optical appearance and color, thermoregulation, wetting characteristics, and flight dynamics.”
Because each scale is made of chitin, “the second most abundant biopolymer on Earth after cellulose,” says Kolle, a new material inspired by butterfly wings could also be biodegradable and easy to change or refresh. He envisions, perhaps, a sprayable version of the scales that could be used on buildings, vehicles or clothing to provide waterproofing and add a little color.
“Either way, imagine how cool that would be, if we’d just had a drum in which we grow the cells that form scales, and then spray them on a surface and supply a trigger that makes the cells grow scales of a desired color, texture, and wetting behavior,” Kolle says.
Fat-Tailed Dwarf Lemurs Hibernate in Captivity for the First Time
/https://tf-cmsv2-smithsonianmag-media.s3.amazonaws.com/filer_public/26/e3/26e3726e-2108-4f40-bcdb-2919b35083fa/lemur_in_blankie.jpeg)
Fat-tailed dwarf lemurs are our closest primate relatives who hibernate in the wild. For the first time ever, researchers were able to recreate the conditions needed to coax the lemurs into hibernation at Duke University’s Lemur Center, which gave them a front row seat to learn about this unique metabolic process—and what it could teach us about humans. Primate behavioral specialist Marina Blanco and her team reported their findings in March in the journal Scientific Reports.
To set the mood for a really good nap, researchers made a makeshift tree hollow for the lemurs to settle into in their enclosure. They exposed the critters to 9.5 hours of light instead of a summery 11 hours to mimic winter’s shortened daylight. They also lowered the enclosure’s temperature to 50 degrees Fahrenheit.
For four months, the sleepy lemurs had dulled metabolisms, cooler body temperatures and much lighter appetites. Their heart rates slowed to eight beats per minute. When they arose again in spring, they bounced right back.
“It is important to remark that hibernators’ metabolic reduction is controlled, meaning that they can tolerate these extremes without ill effects,” says Blanco via email. Despite not moving or eating for months, these animals maintain muscle mass and organ function.
Learning about how another primate hibernates could improve our current methods for slowing down the body’s processes during life-saving surgeries or perhaps even long-term space travel, explains a Duke press release.
“Successful space travel [to distant destinations] will require months or years spent inactive in transit,” Blanco explains. “The closest to the needed suspended animation would be to induce hibernation-like states in astronauts.”
Scientists Decode the "Clap" of Butterfly Wings
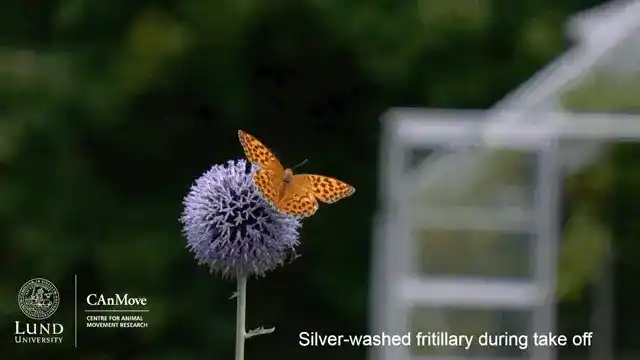
A butterfly is shaped unlike any other flying animal, which makes analyzing their flight all the more exciting and inspiring to scientists. A study published in January 2021 found that butterflies use an efficient cupping and clapping method to generate thrust.
First, a pair of biologists from Lund University, Christoffer Johansson and Per Henningsson, conducted an aerodynamic analysis of free-flying butterflies. From these observations, they found that the broad-winged insects clap their wings together on upstrokes—but the wings don’t press flat together like a pair of hands mid-applause. Instead, butterfly wings bend, which researchers suspected might cup air between them to strengthen their downstroke.
To put their suspicions to the test, the scientists compared the flapping capabilities of two robotic clappers: one with rigid wings and one with flexible wings. They found that flexibility increased wing efficiency by 28 percent and added 22 percent more force to their flaps.
In nature, a butterfly’s wing clap probably gives them the extra boost they need to escape predators. Back in the lab, the pair hope their observations inspire new flying and swimming robots.
“Already today there are flapping drones that clap wings together, such as the DelFly, but they do not use the clap for propulsion, but instead mainly produce forces from the flapping,” Johansson says via email.
Making flexible flapping robots could be a low-energy way to boost thrust. Likewise, the addition of flexibility could be a boon for underwater robotics in cramped environments—perhaps for underwater archaeology.
“A drone designed for slow and precise maneuvering using paddling fins could improve performance by clapping the fins against the body,” he says. “Flexible fins could then create a cupped shape of the fin, similar to what we have seen in the butterfly wings, improving the directionality of the jet produced and the efficiency of the thrust production.”
Tardigrades Have an Insect-Like Walk
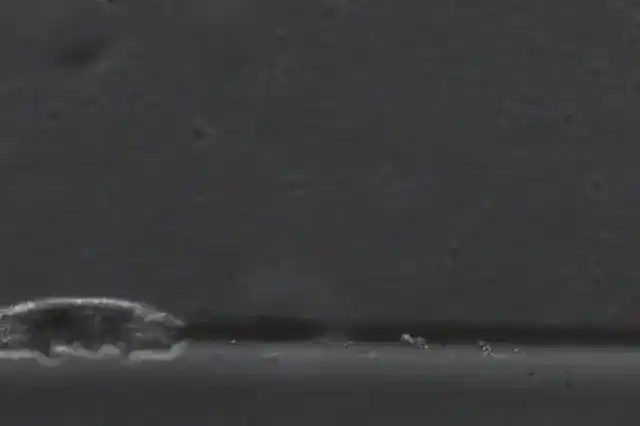
How inspiring are tardigrades? Let us count the ways.
First, they look like tiny, eight-legged, chunky bears—hence their nickname, water bears. These near-microscopic, aquatic extremophiles can survive in the most inhospitable places on Earth, including frigid absolute zero temperatures, boiling hot hydrothermal vents, a vacuum of space, and pressure six times more crushing than the deep ocean, per National Geographic.
While other soft-bodied creatures thrash, slither and wriggle like worms, tardigrades are the only soft-bodied animal that can walk. In a study published in the journal PNAS in August, researchers watched hours of footage of water bears walking on different surfaces from glass to gels.
The team found that tardigrades walk like insects 500,000 times their size. Though they move only half of their already-miniscule body length (0.5 millimeters) per second on average, they can move two body lengths in the same amount of time at top speed. Slow as they may be, they also seem to adjust their steps according to the terrain they’re navigating.
Because their strut has taken them to the far reaches of Earth, studying their gait could inspire new forms of locomotion for micro-scale robots.
“They have some simple, and highly effective ways of coordinating how their eight legs move, and they do this with minimal brain power using some simple rules that are strikingly similar to those used in certain much larger insect species,” says study author Daniel Cohen, a mechanical engineer at Princeton University. “This is great for inspiration because it shows that tiny robots, where computational power and weight are limitations, don’t need much to walk well.”
Tardigrade-inspired nanobots could be injected into damaged tissue to repair it or into an artery to remove plaque build-up—sort of like the shrunken submarine crew in the 1966 sci-fi film Fantastic Voyage.
“And for a completely sci-fi approach: Water bears show us what’s possible at that size when you look like they do. They are sort of like the ‘minimum model’ of what you can do with legs,” Cohen explains. “Perhaps we might someday be able to actually tissue-engineer our own multicellular ‘bio-bots’ made out of living cells and inspired by water bears.”
Slime Mold Stores “Memories” Without Even Having a Brain
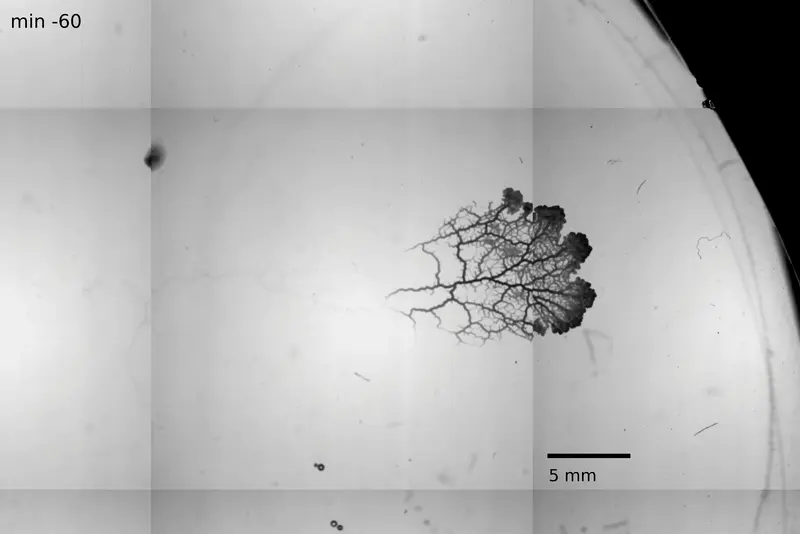
Though they may occasionally resemble fungi, slime molds are globby, soil-dwelling amoebas that come in many odd shapes and bright colors. Despite lacking a brain or nervous system, slime mold can navigate a maze or recall the location of food.
Like gooey fractals, the single-celled organisms unleash tubular tendrils, which also transport fluid and nutrients throughout their sprawling network-like body plan, to explore new environments. In a study published in the journal PNAS in February, researchers found that they record important details of their surroundings by changing the diameter of these outstretched tubes.
When scientists studied a sunny yellow slime mold called Physarum polycephalum under a microscope, they observed the tubes become thicker when they encountered food and thinner when they found none. Some sort of chemical signal likely triggers these changes.
“Given P. polycephalum's highly dynamic network reorganization, the persistence of this imprint sparked the idea that the network architecture itself could serve as memory of the past,” says study author Karen Alim, a biophysicist at the Technical University of Munich, in a statement.
This network sort of mimics how our brain stores memory using synaptic plasticity, meaning synapses become stronger or weaker based on use. Likewise, slime mold tubes that find food grow and dead ends wither away.
Using protein-based polymers, gels or other biological materials, researchers could adapt this sort of memory storage and chemical signaling in artificial intelligence without the need for electronics, Alim explains, which could be a boon for soft robotics that lack such hardware. If the rest of the bot is made using biomaterial, she says, a slime mold–inspired system could be used in situations when toxicity is of concern, like for medical applications or water processing.
“Here, fluid flows are an exciting method to both drive mobility but also encode decision making required for autonomous behavior,” Alim explains via email. “Building the robots out of responsive material that expands in response to the concentration of signaling molecules would here be a direct implementation of the memory mechanism we observed in Physarum.”
With a Soap-Like Protein, Human Cells Can Ward Off Bacteria
Our immune system has specialized cellular soldiers—like T-cells and B-cells—ready to fight off bacterial invasions. However, non-immune cells aren’t entirely defenseless. Research published in Science in July found certain proteins literally have a detergent-like ability to wipe out bacteria like a grease stain. Investigating these proteins further could inspire a totally new kind of antibiotic.
“This is a case where humans make their own antibiotic in the form of a protein that acts like a detergent,” says study author John MacMicking, an immunobiologist at Yale University, in a statement. “We can learn from that.”
First, the team infected non-immune cells with Salmonella, a rod-shaped bacteria that affects the intestines. Then, they screened the cell’s genes for protective proteins and found a match: APOL3. This protein has parts drawn to water and other parts drawn to fats—just like laundry detergent. APOL3 attacks the greasy lipids lining the inner bacterial membrane with precision, never mistaking a human cell membrane for the invader.
As resistance to antibiotics surges, an alternative option is needed. Pathogens may have their own proteins that interfere with APOL3’s counterattacks, but researchers could design a new antibiotic that targets those pesky proteins so APOL3 can do its thing. Cohen also suggests designing small molecules that mimic APOL3’s actions using what’s called host-directed therapy, which creates a harsh environment for the pathogen instead of directly disarming it.
But first, the scientists have to understand the breadth of infections controlled by APOL3 and other immune defense proteins. “That will incentivize the research effort in tackling important human bacterial, viral, and parasitic pathogens, especially if antibiotic resistance is already prevalent for those microbes and we need new drugs,” MacMicking says via email.
“Inventions that mimic host protein activities are still relatively understudied,” MacMicking explains. “This could be fertile ground since our immune defenses are largely indifferent to whether pathogens are currently sensitive or resistant to known antibiotics.”