One Step Closer to Birdflight
NASA’s Spanwise Adaptive Wing program gears up for a second round of test flights.
/https://tf-cmsv2-smithsonianmag-media.s3.amazonaws.com/filer/e8/06/e8068e9b-afb4-4ada-9300-a87aa3339af5/nasa_saw.jpg)
With the Spanwise Adaptive Wing, engineers hope to achieve something Nature figured out long ago.
By changing the vertical angle, or cant, of the outer part of an airplane’s wings—the part closest to the wingtip—in flight, NASA researchers discovered that they could increase fuel efficiency and side-to-side (yaw) stability. It may not sound impressive, but it’s a huge step that could save a lot of energy and take us closer to flying with the grace of birds.
The Spanwise Adaptive Wing project may finally make in-flight wing changes practical. One thing you might notice about birds is that they lack vertical stabilizers, which virtually every aircraft ever built relies on for stability and (thanks to the attached rudder) maneuving in the yaw axis. Airplane tails are designed to cope with the worst possible situations, but much of it simply isn't needed most of the time, according to Matt Moholt, SAW’s principal investigator. “The vertical tail is oversized for the entire flight mission profile,” he says.
Carrying around all that dead weight and extra drag is not ideal in an industry where every pound counts, but nobody has figured out how to both lop off the tail and provide yaw authority—until the SAW tests. The SAW team proved the concept feasible over three flight tests in late 2017/early 2018 on a 30 percent-scale 737, dubbed Prototype-Technology Evaluation and Research Aircraft (PTERA).
They found that canting the wings 45 degrees upwards provided enough yaw, but decreased lateral stability. Canting the wings down, on the other hand, provided stability but not much yaw. So an airplane capable of changing the cant of its wings inflight could get yaw when it’s needed, then shift to get more stability when it’s not.
“Can we generate enough yaw control from the wing tips to help remove or reduce the vertical tail? On the PTERA UAV we find that the answer is yes,” says Moholt. “In fact, our next flight test campaign seeks to demonstrate this. The flight test data from the first campaign shows that we only need to deflect the wings up 40 degrees to gain enough yaw to eliminate a significant portion of the rudder.”
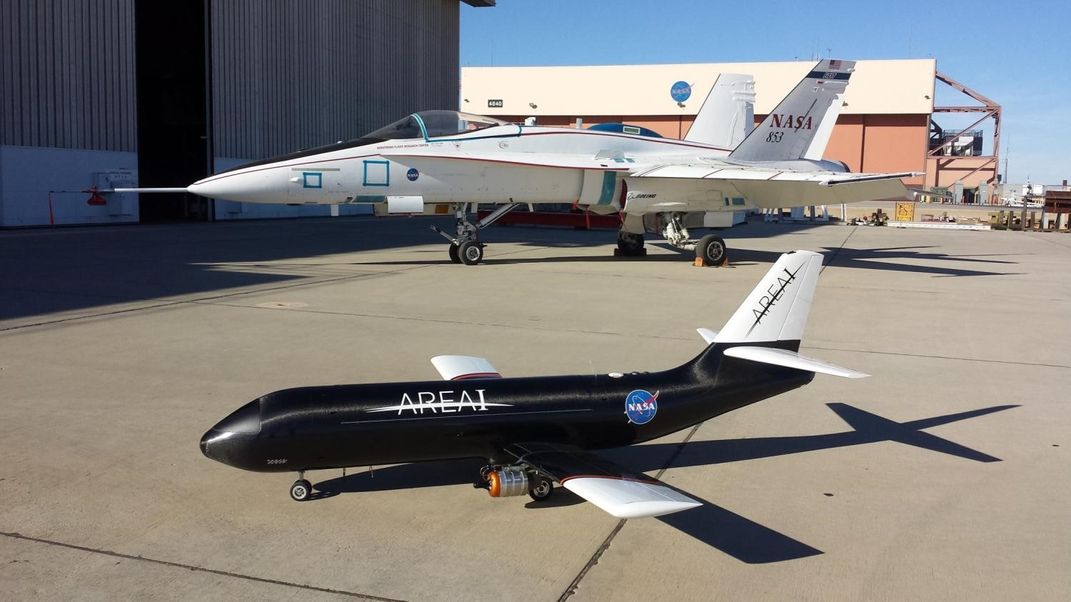
SAW is possible thanks to a technology—shape-memory alloys—that wasn't available during the golden age of flight testing. These alloys expand in a predictable, directed way when exposed to high heat, so they can be much smaller and less complex than the large and sturdy control mechanisms used now.
Using shape-memory alloys for this purpose is new, so for now the SAW team is focused on making sure it works, rather than optimizing its efficiency, says Othmane Benafan, co-principal investigator and lead materials engineer for the project. Future experiments could swap out the dedicated battery system that now heats the alloy and use cartridge or induction heaters, which are more efficient, instead.
The team is experimenting with memory alloys on an F/A-18 wing, but for the moment has no immediate plans to fly on such a big jet. “The PTERA UAV test flight was on a 200-pound vehicle, so moving straight to a 25,000-pound F/A-18 flight test is too much of a leap,” says Othmane. “We plan an intermediate step to test on a roughly 2000-pound vehicle.”
The next round of PTERA flights, a month or two from now, will also fine-tune the wing-cant for fuel efficiency. “For our second flight test campaign, we will be flying an experimental control system which monitors fuel flow and is constantly moving the wing tips to find the optimal position from an efficiency perspective,” says Moholt.
Artificial wings have never been ideal, and in comparison to birds, airplanes are awkward, ungraceful fliers. Birds easily and precisely morph and move their wings, and as a result can fly with an ease and flexibility that turn aeronautical engineers green with envy. Since metal (and increasingly, carbon-fiber) wings can’t morph, every airplane wing is a careful compromise between what designers want it to do best and what it needs to do the rest of the time. Most commercial airline wings are carefully refined for high-altitude, high-speed cruise, and flying slowly for takeoff and landing requires peripheral changes—flaps and slats, spoilers and speedbrakes—whose function any bird would recognize but would find unbearably clumsy. A 737 requires thousands of feet of concrete to take off, but even the fastest birds can morph their wings such that a quick hop or very short run gives them enough lift to fly.
Not that we haven’t tried emulating birds. The Wright Brothers’ airplanes turned by warping the wooden-frame, cloth-covered wings, contorting them to increase lift on one and decrease on the other. (That method was very quickly replaced by ailerons, hinged panels on the wing’s trailing edge, which are easier and simpler to build). Swing-wings were the rage among 1960s military jet designers—many birds also tuck their wings in for speed—but they were complex and maintenance-intensive, and never left the concept stage in the civilian world.
Seems there’s still plenty to be learned from studying the biology of flight.