A New Wireless Brain Implant Helps Paralyzed Monkeys Walk. Humans Could Be Next.
One small step for monkeys, one potential leap for humans
:focal(2373x1268:2374x1269)/https://tf-cmsv2-smithsonianmag-media.s3.amazonaws.com/filer/55/3b/553b7f79-9fdc-4338-8a3f-185d033e780a/img_8687_dxo.jpg)
The two paralysis patients were up and walking on treadmills in no time. This impressive feat was made possible by an unprecedented new surgery, in which researchers implanted wireless devices in the patients’ brains that recorded their brain activity. The technology allowed the brain to communicate with the legs—bypassing the broken spinal cord pathways—so that the patient could once again regain control.
These patients, it turns out, were monkeys. But this small step for monkeys could lead to a giant leap for millions of paralyzed humans: The same equipment has already been approved for use in humans, and clinical studies are underway in Switzerland to test the therapeutic effectiveness of the spinal cord stimulation method in humans (minus the brain implant). Now that researchers have a proof-of-concept, this kind of wireless neurotechnology could change the future of paralysis recovery.
Instead of trying to repair the damaged spinal cord pathways that usually deliver brain signals to the limbs, scientists tried an innovative approach to reverse paralysis: Bypassing the injury bottleneck altogether. The implant worked as a bridge between the brain and the legs, directing leg motion and stimulating muscle movement in real time, says Tomislav Milekovic, a researcher at Switzerland's École Polytechnique Fédérale de Lausanne (EPFL). Milekovic and co-authors report their findings in a new paper published Wednesday in the journal Nature.
When the brain's neural network processes information, it produces distinctive signals—which scientists have learned to interpret. Those that drive walking in primates originate in the dime-sized region known as the motor cortex. In a healthy individual, the signals travel down the spinal cord to the lumbar region, where they direct the activation of leg muscles to enable walking.
If a traumatic injury severs this connection, a subject is paralyzed. Although the brain is still able to produce the proper signals, and the leg's muscle-activating neural networks are intact, those signals never reach the legs. The researchers managed to reestablish the connection thorough real-time, wireless technology—an unprecedented feat.
How does the system work? The team's artificial interface begins with an array of almost 100 electrodes implanted in the brain's motor cortex. It's connected to a recording device that measures the spiking of electrical activities in the brain that control leg movements. The device sends these signals to a computer that decodes and translates these instructions to another array of electrodes implanted in the lower spinal cord, below the injury. When the second group of electrodes receives the instructions, it activates the appropriate muscle groups in the legs.
For the study, the two Rhesus macaque monkeys were given spinal cord injuries in the lab. After their surgeries, they had to spend a few days recovering and waiting for the system to collect and calibrate necessary data on their condition. But just six days after injury, one monkey was walking on a treadmill. The other was up and walking on post-injury day 16.
The success of the the brain implant demonstrates for the first time how neurotechnology and spinal cord stimulation can restore a primate's ability to walk. “The system restored locomotor movements immediately, without any training or re-learning,” Milekovic, who engineers data-driven neuroprosthetic systems, told Smithsonian.com.
“The first time we turned the brain-spine interface on was a moment that I’ll never forget,” added EPFL researcher Marc Capogrosso in a statement.
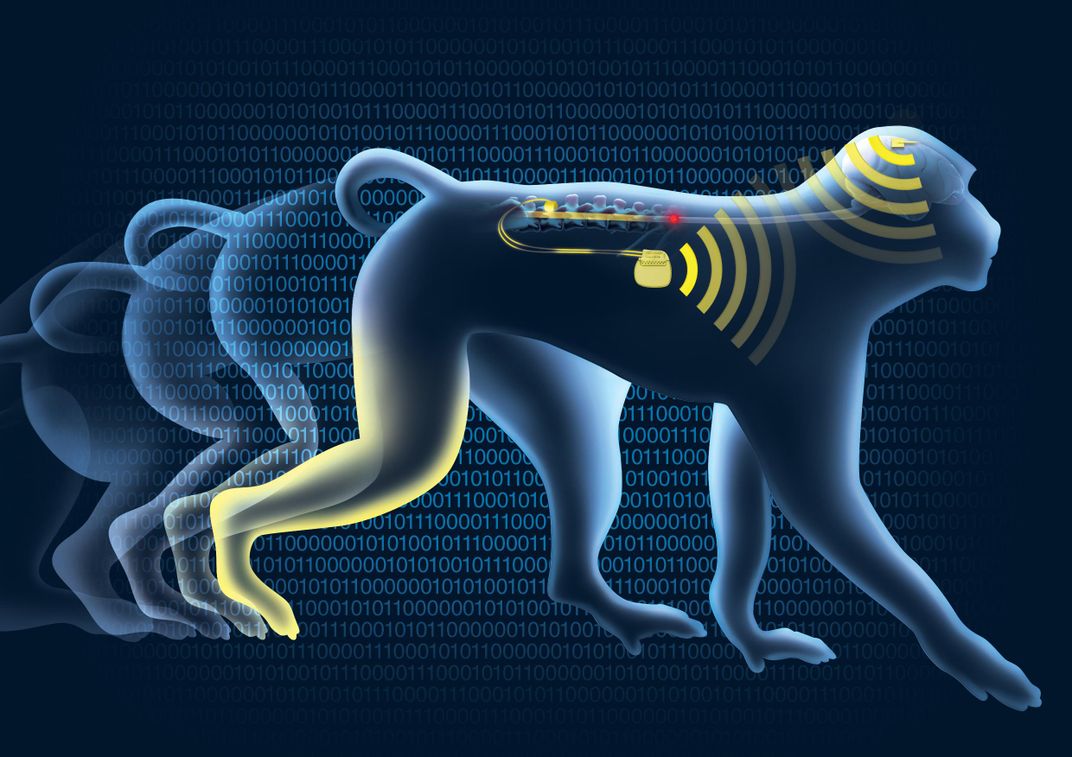
The technique of "hacking" the brain's neural networks has produced remarkable feats, such as helping to create touch-sensitive prosthetics that allow wearers to perform delicate tasks like cracking an egg. But many of these efforts use cable connections between the brain and recording devices, meaning the subjects aren't able to move freely. “Neural control of hand and arm movements was investigated in great detail, while less focus has been given to the neuronal control of leg movements, which required animals to move freely and naturally,” Milekovic says.
Christian Ethier, a neuroscientist at Quebec's Université Laval who was not involved in the research, called the work a “major step forward in the development of neuroprosthetic systems." He added: “I believe this demonstration is going to accelerate the translation of invasive brain-computer interfaces toward human applications.
In an accompanying News & Views piece in Nature, neuroscientist Andrew Jackson agrees, pointing out how quickly advances in this field have moved from monkeys to people. A 2008 paper, for instance, demonstrated that paralyzed monkeys could control a robotic arm with just their brain; four years later a paralyzed woman did the same. Earlier this year, brain-controlled muscle stimulation enabled a quadriplegic person to grasp items, among other practical hand skills, after the same feat was achieved in monkeys in 2012.
Jackson concludes from this history that “it's not unreasonable to speculate that we could see the first clinical demonstrations of interfaces between the brain and spinal cord by the end of of the decade.”
The Blackrock electrode array implanted in the monkeys' brains has been used for 12 years to successfully record brain activity in the BrainGate clinical trials; numerous studies have demonstrated that this signal can accurately control complex neuroprosthetic devices. “While it does require surgery, the array is an order of magnitude smaller than the surgically implanted deep brain simulators already used by more than 130,000 people with Parkinson's disease or other movement disorders,” Milekovic adds.
While this test was limited to just a few phases of brain activity related to walking gait, Ethier suggests that it could potentially enable a greater range of movement in the future. “Using these same brain implants, it is possible to decode movement intent in a lot more detail, similar to what we have done to restore grasp function. ... I expect that future developments will go beyond and perhaps include other abilities like compensating for obstacles and adjusting walking speed.”
Ethier notes another intriguing possibility: The wireless system might actually help the body heal itself. “By re-synchronizing the activity in the brain and spinal motor centers, they could promote what is called ‘activity-dependent neuroplasticity,’ and consolidate any spared connections linking the brain to the muscles,” he says. “This could have long-term therapeutic effects and promote the natural recovery of function beyond what is possible with conventional rehabilitation therapies.”
This phenomenon is not well understood, and the possibility remains speculative at this point, he stresses. But the tangible achievement this research demonstrates—helping the paralyzed walk again with their brains—is already a huge step.