Why Does Cardiac Arrest Often Strike in the Morning?
Studies show that the amount of a specific molecule in human hearts fluctuates on a daily cycle, helping to explain the decades-old observation
/https://tf-cmsv2-smithsonianmag-media.s3.amazonaws.com/filer/20130908102129heartelectric2.jpg)
For decades scientists have known that sudden cardiac death–a failure in the heart’s electrical system that leads people to, well, suddenly drop dead–occurs more often in the morning hours. Analysis of data from the ambitious Framingham Heart Study led to the scientific documentation of the curious link as early as 1987. But for just as long, scientists haven’t been able to do much with that knowledge. A flurry of papers in the late 1980s pointed to possible explanations: the assumption of an upright posture, for example, or problems with the process that typically prevents blood clots. Still, scientists have been unable to pin down a basic mechanism to explain the connection between the body’s circadian clock and the electrical mishap that causes sudden death.
Now an international team of researchers has stumbled upon a lead. Mukesh Jain of Case Western Reserve University in Cleveland and his colleagues recently identified a protein whose levels oscillate with the circadian clock and, in mice, cause the ion channels governing the heart’s electrical system to oscillate with the clock too. On September 8 in Indianapolis at a meeting of the American Chemical Society (ACS), Jain reported that these oscillations also occur in human heart cells. The results point to an era when doctors may be capable of preventing sudden cardiac death, which is the leading cause of natural death in the United States, killing more than 300,000 people each year.
To understand the ins and outs of Jain’s finding, one first needs to understand how the heart works. Think: car engine, says James Fang, the chief of cardiovascular medicine at the University of Utah School of Medicine in Salt Lake City. There’s the circulating blood, which is the fuel. There are the muscles, which pump that fuel. And there is an electrical system, with charge separation created not by a battery but by ion pumps and ion channels. Without a working electrical system, the muscles won’t expand and contract and the blood won’t flow. In a heart attack, the flow of fuel to the heart is blocked. But in sudden cardiac death, there is an electrical malfunction that prevents the heart from properly pumping blood to the body and brain. The heart’s beating becomes erratic, often displaying a type of arrhythmia called ventricular fibrillation. Heart attacks can lead to the kind of arrhythmia that can lead to sudden cardiac death, but in other cases there is no obvious trigger. No matter how the heart’s plug is pulled, death typically occurs within minutes.
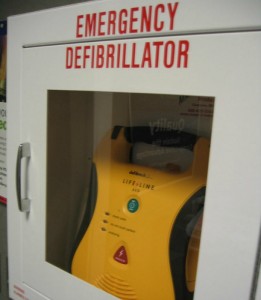
Emergency defibrillators in public places save lives by offering a quick way to shock the heart into working again. But new research on the circadian rhythms of protein found in human hearts may offer a better solution. Photo by Olaf Gradin via flickr
Though drugs for the heart do exist–think beta blockers, ACE inhibitors–there is no drug that acts specifically to prevent the onset of arrhythmia. The most common medical response is just that: a response. Doctors treat the electrical malfunction after it has happened with a defibrillator, a technology with a history stretching back to the end of the 19th century. In 1899, two physiologists found that electrical shocks could not only create but also stop rhythmic disturbances in the heart of a dog. By the end of the 1960s, cardiac defibrillation was being reliably used on people. And in 1985, a Johns Hopkins University doctor got FDA approval for an implantable defibrillator.
Defibrillation has been the primary solution for life-threatening arrhythmias since. These devices have shrunk from “the size of luggage to the size of a cigarette box,” says Fang, and automated external versions have become popular so bystanders can help a victim without the delay of an ambulance ride. But, “it’s a bit of a crude approach,” Fang says. “Defibrillators have really formed the cornerstone for the past two or three decades, but it is not really much of a management solution,” he adds. “It is not preventing the problem. It is letting it happen and then shocking you out of it.” It’s the equivalent of jump-starting a car after the battery has died.
What’s more, Fang says, because scientists don’t know what triggers the arrhythmia to begin with, it’s tough to predict who needs a defibrillator. Take, say, 100 patients who all have weak hearts. “Probably only 10 are going to die suddenly. We don’t know who those 10 are, so we give defibrillators to all 100 people,” Fang says. “It is overkill because 90 don’t even need it. But I can’t tell which 10 are going to die.”
Here’s where Jain’s work comes in. His team, which has long studied a protein known as KLF15, serendipitously discovered that the amount of the protein in a mouse’s heart tissue cycles–going from low to high and back again over a 24-hour period. Though Jain doesn’t study electrophysiology specifically, he was aware of the link between the clock and sudden cardiac death, and he wondered whether his protein (which had been previously connected to some heart diseases) might play a role. Jain’s team found that levels of KLF15 should be high during transitions from night to day, but instead are low in mice that experience sudden cardiac death–suggesting their hearts don’t have enough of the protein during a crucial window. KLF15 controls the levels of another protein that affects how ions flow into and out of the mouse’s heart, meaning the ion channels also follow a circadian rhythm. When the researchers eliminated the presence of KLF15, “the ion channel expression went down and didn’t oscillate,” Jain says. “And these animals had increased susceptibility to ventricular arrhythmias and sudden death.” The study was published last year in Nature.
Follow-up observations, presented at the ACS meeting, confirm that the oscillation of KLF15 and the ion channels occur in human heart cells. Those findings “start to build a case that this is potentially important to human biology and human disease,” Jain says.
Jain believes his molecular work and other similar studies on the horizon could lead to drugs that offer a solution better than defibrillation. “We need a fresh start,” he says. “What we are doing ain’t working.” But there is a long way still to go. Future studies will try to find molecules that could boost KLF15 levels, to look for other clock-related molecules at work in the heart and to seek out genetic variants associated with sudden cardiac death.