What Dung Beetles Can Teach Us About Sexual Difference
When it comes to sex appeal, it’s not all in your genes (it’s also in your proteins!)
/https://tf-cmsv2-smithsonianmag-media.s3.amazonaws.com/filer/40/9f/409fe494-f4eb-4d6a-bb16-8b3c11d6912f/cfntac.jpg)
Picture a lion: The male has a luxuriant mane, the female doesn’t. This is a classic example of what biologists call sexual dimorphism—the two sexes of the same species exhibit differences in form or behavior. Male and female lions pretty much share the same genetic information, but look quite different.
We’re used to thinking of genes as responsible for the traits an organism develops. But different forms of a trait—mane or no mane—can arise from practically identical genetic information. Further, traits are not all equally sexually dimorphic. While the tails of peacocks and peahens are extremely different, their feet, for example, are pretty much the same.
Understanding how this variation of form—what geneticists call phenotypic variation—arises is crucial to answering several scientific questions, including how novel traits appear during evolution and how complex diseases emerge during a lifetime.
So researchers have taken a closer look at the genome, looking for the genes responsible for differences between sexes and between traits within one sex. The key to these sexually dimorphic traits appears to be a kind of protein called a transcription factor, whose job it is to turn genes “on” and “off.”
In our own work with dung beetles, my colleagues and I are untangling how these transcription factors actually lead to the different traits we see in males and females. A lot of it has to do with something called “alternative gene splicing”—a phenomenon that allows a single gene to encode for different proteins, depending on how the building blocks are joined together.
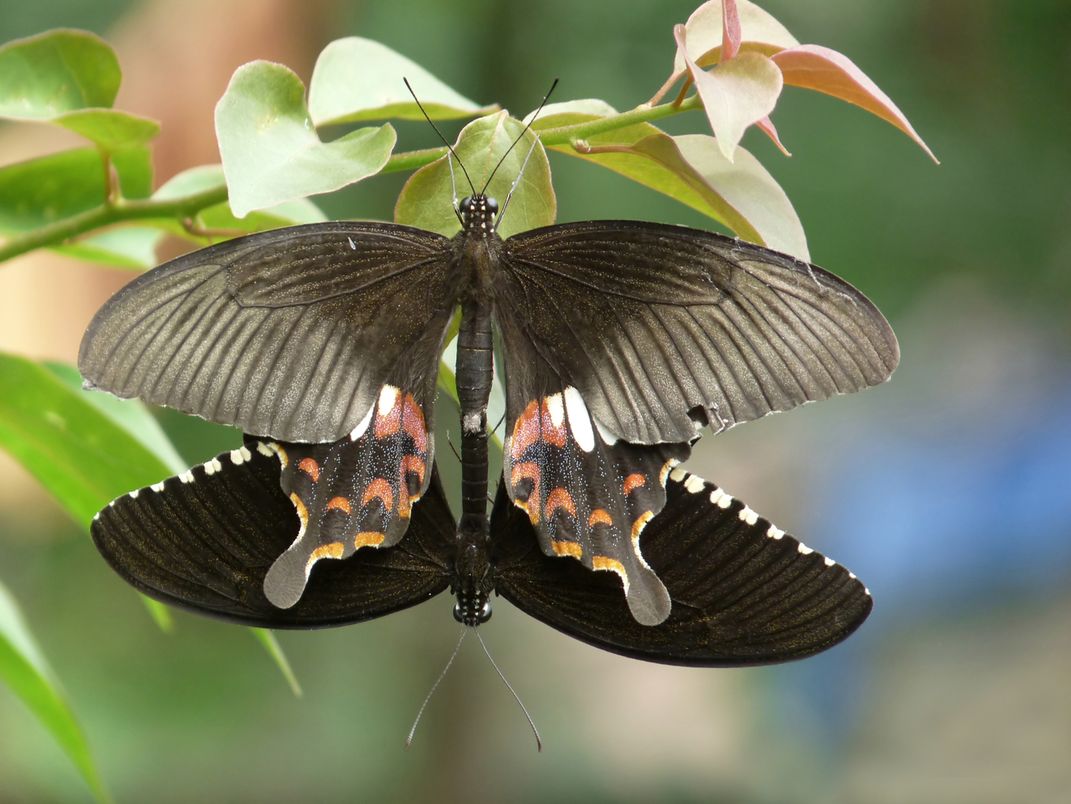
Over the years, different groups of scientists independently worked with various animals to identify genes that shape sexual identity; they realized that many of these genes share a specific region. This gene region was found in both the worm gene mab-3 and the insect gene doublesex, so they named similar genes containing this region DMRT genes, for “doublesex mab-related transcription factors.”
These genes code for DMRT proteins that turn on or off the reading, or expression, of other genes. To do this, they seek out genes in DNA, bind to those genes, and make it either easier or harder to access the genetic information. By controlling what parts of the genome are expressed, DMRT proteins lead to products characteristic of maleness or femaleness. They match the expression of genes to the right sex and trait.
DMRTs almost always confer maleness. For instance, without DMRT, testicular tissue in male mice deteriorates. When DMRT is experimentally produced in female mice, they develop testicular tissue. This job of promoting testis development is common to most animals, from fish and birds to worms and clams.
DMRTs even confer maleness in animals where individuals develop both testes and ovaries. In fish that exhibit sequential hermaphroditism—where gonads change from female to male, or vice versa, within the same individual—the waxing and waning of DMRT expression results in the appearance and regression of testicular tissue, respectively. Likewise, in turtles that become male or female based on temperatures experienced in the egg, DMRT is produced in the genital tissue of embryos exposed to male-promoting temperatures.
The situation is a little different in insects. First, the role of DMRT (doublesex) in generating sexual dimorphism has extended beyond gonads to other parts of the body, including mouthparts, wingspots and mating bristles aptly named “sex combs.”
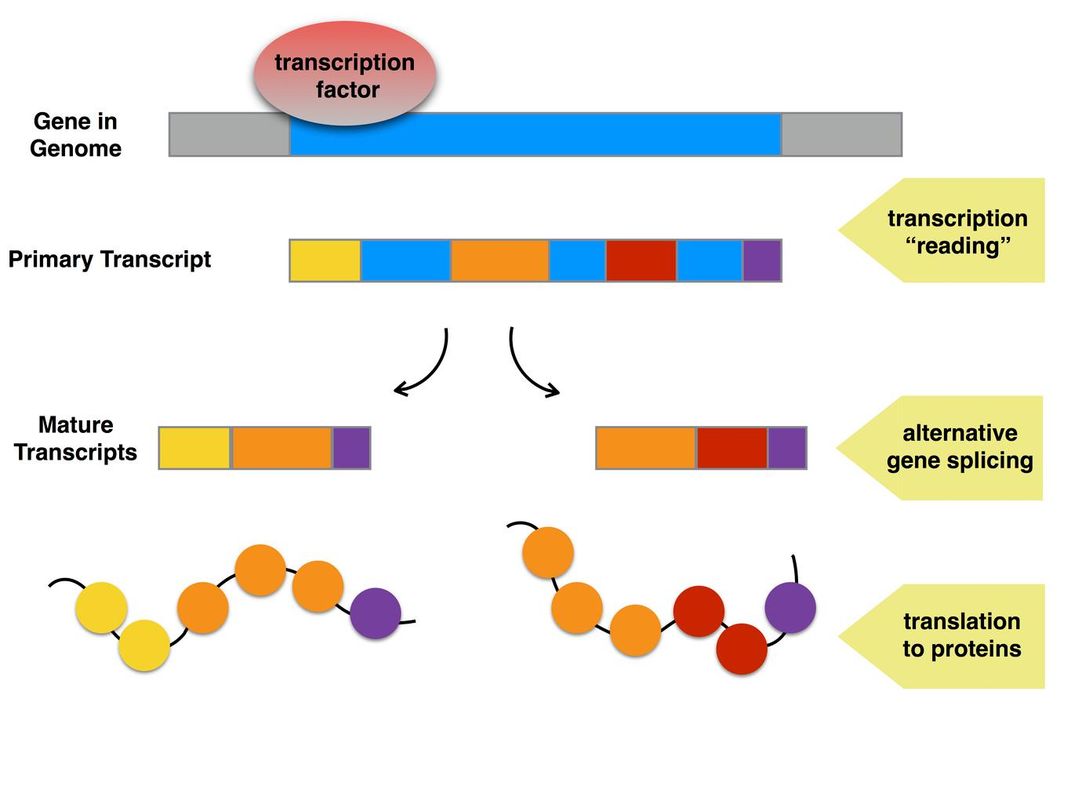
Secondly, male and female insects generate their own versions of the doublesex protein through what’s called “alternative gene splicing.” This is a way for a single gene to code for multiple proteins. Before genes are turned into proteins, they must be turned “on”; that is, transcribed into instructions for how to build the protein.
But the instructions contain both useful and extraneous regions of information, so the useful parts must be stitched together to create the final protein instructions. By combining the useful regions in different ways, a single gene can produce multiple proteins. In male and female insects, it’s this alternative gene splicing that results in the doublesexproteins behaving differently in each sex.
So in a female, instructions from the doublesex gene might include sections 1, 2 and 3, while in a male the same instruction might include only 2 and 3. The different resulting proteins would each have their own effect on what parts of the genetic code are turned on or off—leading to a male with huge mouthparts and a female without, for instance.
How do male and female forms of doublesex regulate genes to produce male and female traits? Our research group answered this question using dung beetles, which are exceptionally numerous in species (over 2,000), widespread (inhabiting every continent except Antarctica), versatile (consuming about every type of dung) and show amazing diversity in a sexually dimorphic trait: horns.
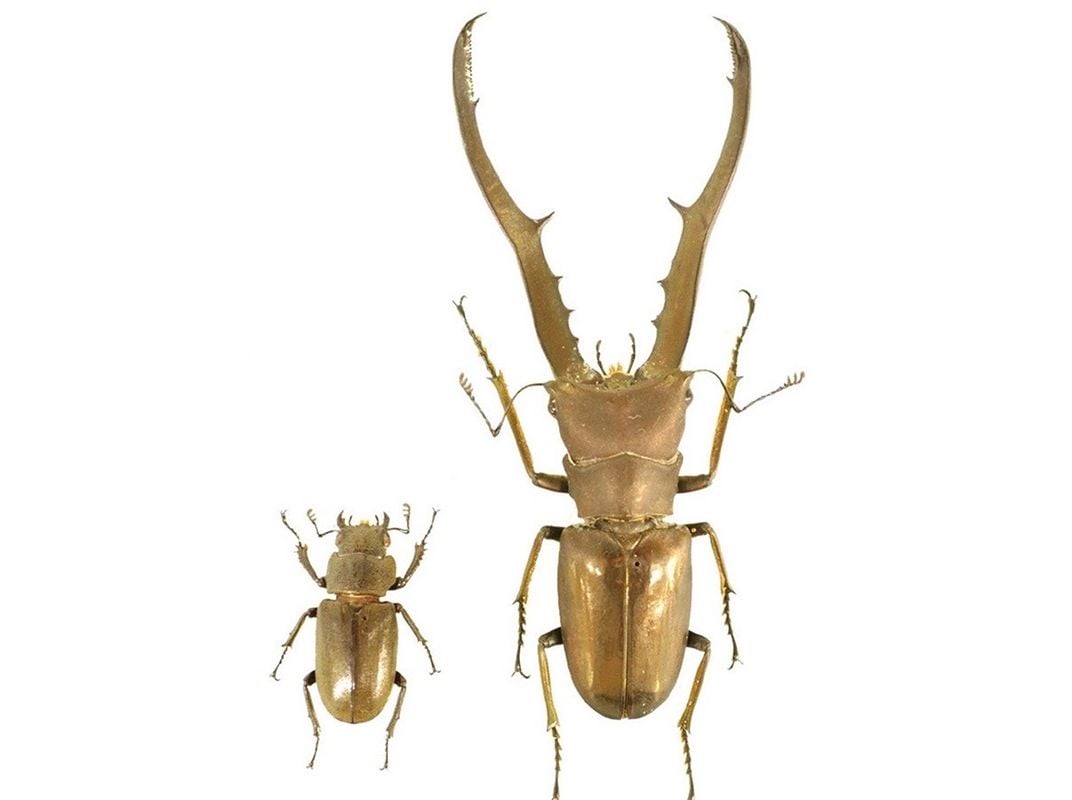
We focused on the bull-headed dung beetle Onthophagus taurus, a species in which males produce large, bull-like head horns but females remain hornless. We found that doublesex proteins can regulate genes in two ways.
In most traits, it regulates different genes in each sex. Here, doublesex is not acting as a “switch” between two possible sexual outcomes, but instead bestowing maleness and femaleness to each sex independently. Put another way, these traits don’t face a binary decision between becoming male or female, they are simply asexual and poised for further instruction.
The story is different for the dung beetles’ head horns. In this case, doublesex acts more like a switch, regulating the same genes in both sexes but in opposite directions. The female protein suppressed genes in females that would otherwise be promoted by the male protein in males. Why would there be an evolutionary incentive to do this?
Our data hinted that the female doublesex protein does this to avoid what is known as “sexual antagonism.” In nature, fitness is sculpted by both natural and sexual selection. Natural selection favors traits increasing survival, whereas sexual selection favors traits increasing access to mates.
Sometimes these forces are in agreement, but not always. The large head horns of male O. taurus increase their access to mates, but the same horns would be a hassle for females who have to tunnel underground to raise their offspring. This creates a tension between the sexes, or sexual antagonism, that limits the overall fitness of the species. However, if the female doublesex protein turns “off” genes that in males are responsible for horn growth, the whole species does better.
Our ongoing research is addressing how doublesex has evolved to generate the vast diversity in sexual dimorphism in dung beetles. Across species, horns are found in different body regions, grow differently in response to different quality diets, and can even occur in females rather than males.
In Onthophagus sagittarius, for instance, it’s the female that grows substantial horns while males remain hornless. This species is only five million years diverged from O. taurus, a mere drop of time in the evolutionary bucket for insects. For perspective, beetles diverged from flies about 225 million years ago. This suggests that doublesex can evolve quickly to acquire, switch, or modify the regulation of genes underlying horn development.
How will understanding the role of doublesex in sexually dimorphic insect traits help us understand phenotypic variation in other animals, even humans?
Despite the fact that DMRTs are spliced as only one form in mammals and act primarily in males, the majority of other human genes are alternatively spliced; just like insects’ doublesex gene, most human genes have various regions that can be spliced together in different orders with varying results. Alternatively spliced genes can have distinct or opposing effects based on which sex or trait they’re expressed in. Understanding how proteins that are produced by alternatively spliced genes behave in different tissues, sexes and environments will reveal how one genome can produce a multitude of forms depending on context.
In the end, the humble dung beetle’s horns can give us a peek into the mechanisms underlying the vast complexity of animal forms, humans included.
This article was originally published on The Conversation.

Cris Ledón-Rettig, Postdoctoral Fellow of Biology, Indiana University, Bloomington