After a Century of Searching, We Finally Detected Gravitational Waves
Two merging black holes sent out a signal 1.3 billion years ago that now confirms a key prediction of Einstein’s relativity
/https://tf-cmsv2-smithsonianmag-media.s3.amazonaws.com/filer/84/84/84840adb-66dc-4967-bdfc-616ca72ab32a/146977main_gwave_lg4.jpg)
Scientists have heard gravity’s aria for the first time.
As two black holes spiraled toward each other and merged, they created ripples in the fabric of the cosmos in exactly the form physicists have predicted for a century: gravitational waves. Unveiled today during a suite of international press conferences, the signal paves the way for a whole new understanding of the universe.
"This is the first time the universe has spoken to us through gravitational waves. Up until now we have been deaf," LIGO Laboratory Director David Reitze, of the University of Florida, said today at a press event in Washington, D.C.
At the root of gravitational waves is Albert Einstein’s theory of gravity, which says that anything with mass warps the very fabric of space-time. When massive objects move, they create distortions in the cosmic fabric, generating gravitational waves. These waves ripple through the universe like sound waves pulsing through the air.
Einstein's theory predicts that the universe is teeming with gravitational waves, but until now we hadn’t been able to detect them, in part because the waves are exceptionally faint. But even before its upgraded instruments came officially online last year, the Laser Interferometer Gravitational-Wave Observatory (LIGO) picked up a clear signal from the powerful collision of two black holes 1.3 billion light-years away.
“To have a gravitational wave signal detected while LIGO is still not near design sensitivity in the first science run is astonishing, it’s jaw-dropping, in a good way” says Joan Centrella, who headed up the Gravitational Astrophysics Laboratory at NASA's Goddard Space Flight Center before becoming the deputy director of the Astrophysics Science Division at Goddard.
That exhilaration rippled through LIGO’s Livingston, Louisiana, observatory and through the rest of the world as the team made their announcement. Nearly everything that astronomers have learned about the cosmos has come from different forms of light, such as visible, radio waves and X-rays. But just as seismic waves can reveal hidden structures deep inside Earth, gravitational waves carry with them information about hidden properties of the universe that even light can't reveal.
“We began with a high-risk job with a very high potential payoff,” Kip Thorne, a LIGO co-founder and a gravitational physicist at the California Institute of Technology, said during the press event. “And we are here today with a great triumph—a whole new way to observe the universe.”
Early Clues
The hunt for gravitational waves began a century ago, with the publication of Einstein’s general theory of relativity. In the mid-1970s, physicists Russell A. Hulse and Joseph H. Taylor, Jr. captured extremely convincing evidence that these ripples exist. They measured the time it took for two dense neutron stars—the crushed cores of once-massive stars—to orbit each other.
Based on Einstein's work, they knew these stars should be radiating gravitational energy as they spun, and that lost energy should cause them to spiral towards each other. After studying the two stars for the next few years, they saw that the orbit decreased by exactly the amount predicted by general relativity.
While that finding earned the duo the 1993 Nobel prize in physics, most physicists wouldn’t call it a direct detection of gravitational waves.
In 2001, LIGO began operating at two locations 1,875 miles apart—one in Livingston, Louisiana and the other in Hanford, Washington. A few years later, the European gravitational-wave telescope Virgo also came online. Both operated until 2010 and 2011, respectively, before going offline for upgrades.
While scientists had hoped these initial observatories would capture gravitational waves, they knew it was a long shot. These ripples are very weak signals, and the instruments weren’t sensitive enough to hear their whispers. But the initial runs serves as tests of the technology for the next-generation instruments.
Virgo is still being upgraded, but the LIGO team completed their work on both detectors in 2015. Now called Advanced LIGO, the Louisiana and Washington observatories listened for gravitational waves during the first science-observing run between September 18, 2015, and January 12, 2016. The signal announced today was picked up just prior to that first official run, as the team was running operational tests of the detectors.
Laser Precision
Sensing a wave as it passed through Earth required a lot of clever engineering, computer power and more than 1,000 scientists working around the world.
Inside each L-shaped LIGO observatory, a laser sits at the meeting point of two perpendicular tubes. The laser passes through an instrument that splits the light, so that two beams travel the roughly 2.5 miles down each tube. Mirrors at the ends of the tubes reflect the light back towards its source, where a detector waits.
Typically no light lands on the detector. But when a gravitational wave passes though, it should stretch and squish space-time in a predictable pattern, effectively changing the lengths of the tubes by a tiny amount—on the order of one-thousandth the diameter of a proton. Then, some light will land on the detector.
To account for the incredibly small change, the instrument's mirrors are attached to complex systems that isolate them from most vibrations. LIGO scientists also have special computer programs that can filter through various sorts of background noise, like occasional tremors, and determine if any incoming signal matches possible astronomical sources calculated using general relativity.
The Louisiana and Washington sites work together to verify a sighting. “We don’t believe that we see a gravitational wave unless both detectors see the same signal within the amount of time that the gravitational wave would take to travel between the two sites,” says LIGO team member Amber Stuver of Louisiana State University. In this case, the wave passed through Earth and hit the two detectors just seven milliseconds apart.
Once the Louisiana and Washington sites detect a possible gravitational tune, scientists get to work on the analysis. LIGO picked up this signal on September 14 but is only now able to say with high certainty that they saw gravitational waves.
"It took us months of careful checking, re-checking, analysis, working with every piece of data to make sure of the sighting," Reitze said during the D.C. event. "And we've convinced ourselves that is the case." The results appear this week in Physical Review Letters.
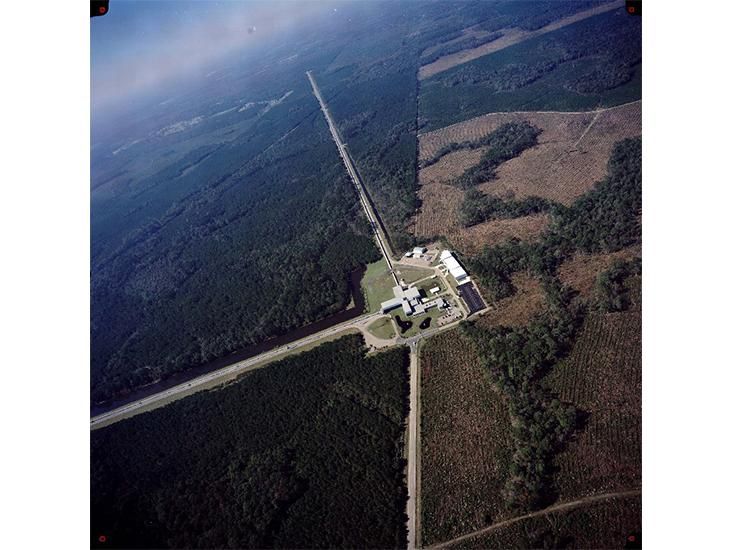
The gravitational wave signal that astronomers pulled out of the most recent observations matched what they expected for two black holes spiraling toward each other. The dance sends out gravitational waves at a predictable frequency and strength, depending on how far apart the objects are and on their masses.
As they begin to dance closer, the wavelengths of the gravitational waves shrink and their song reaches higher pitches. When the black holes close in for the final embrace, the gravitational wave signal has one final high note, or “chirp,” as astronomers call it.
The September signal lines up beautifully with what the team would expect from two black holes with masses equal to about 29 and 36 times the mass of the sun. Those black holes slammed together to create a new black hole 62 times the mass of the sun—radiating away 3 solar masses worth of gravitational energy.
Expect the Unexpected
With this initial detection, astronomers are hopeful that Advanced LIGO will continue to capture gravitational waves and start building up data for all kinds of scientific studies, from figuring out how supernovas work to learning about the universe’s first few moments. While no other astronomical telescope saw any sign of this black hole collision, some of the other sources Advanced LIGO is looking for should have counterparts visible to telescopes that capture light.
This seems especially promising considering that Advanced LIGO is not even at its full sensitivity yet. That will come in the next few years, says Stuver.
Each of these signals will give astronomers what they never had before: a way to probe extreme cases of gravity and the movements of invisible objects. Even more exciting, astronomers know that with each technological advance, the universe has a way of surprising us.
“Every time that we’ve looked in a new way and different kind of light, we discover something we didn’t expect to find," says Stuver. "And it’s that unexpected thing that revolutionizes our understanding of the universe.” Not long after astronomers turned radio antennas on the sky, they discovered an unexpected type of neutron star called a pulsar. And, perhaps poetically, it was a pulsar and neutron star doing an orbital dance that Hulse and Taylor studied in the 1970s.
Now, with the dawn of gravitational-wave astronomy, scientists have a new tool for sampling the cosmos. And from the sound of it, we’re in for some beautiful music.
Editor's Note: Joan Centrella's affiliation has been corrected.