Destination: Moon or Asteroid? Part III: Resource Utilization Considerations
/https://tf-cmsv2-smithsonianmag-media.s3.amazonaws.com/filer/20111212122020asteroid-mining-intro-300x225.jpg)
Part III: Resource Utilization Considerations
In Part I and Part II of this series, I examined some of the operational and scientific issues associated with a human mission to a near Earth asteroid (NEO) and contrasted them with the simpler operations and greater scientific return of a mission to the Moon. To continue the discussion of what we might do at an asteroid, I will now consider using the local resources offered by asteroids, how they differ from those of the Moon, and offer some practical considerations on accessing and using them.
To become a truly space faring species, humanity must learn how to use what we find in space to survive and thrive. Tied to the logistics chain of the Earth, we are now and always will be limited in space capability. Our ultimate goal in space is to develop the capability to go anywhere at any time and conduct any mission we can imagine. Such capability is unthinkable without being able to obtain provisions from resources found off-planet. That means developing and using the resources of space to create new capabilities.
One of the alleged benefits of asteroid destinations is that they are rich in resource potential. I would agree, putting the accent on the word “potential.” Our best guide to the nature of these resources comes from the study of meteorites, which are derived from near Earth asteroids. They have several compositions, the most common being the ordinary chondrite, which makes up about 85% of observed meteorite falls. Ordinary chondrites are basically rocks, rich in the elements silicon, iron, magnesium, calcium and aluminum. They contain abundant metal grains, composed mostly of iron and nickel, widely dispersed throughout the rock.
The resource potential of asteroids lies not in these objects, but in the minority of asteroids that have more exotic compositions. Metal asteroids make up about 7% of the population and are composed of nearly pure iron-nickel metal, with some inclusions of rock-like material as a minor component. Other siderophile (iron-loving) elements including platinum and gold make up trace portions of these bodies. A metal asteroid is an extremely high-grade ore deposit and potentially could be worth billions of dollars if we were able to get these metals back to Earth, although one should be mindful of the possible catastrophic effects on existing precious metal markets – so much gold was produced during the 1849 California Gold Rush that the world market price of gold decreased by a factor of sixteen.
From the spaceflight perspective, water has the most value. Another type of relatively rare asteroid is also a chondrite, but a special type that contains carbon and organic compounds as well as clays and other hydrated minerals. These bodies contain significant amounts of water. Water is one of the most useful substances in space – it supports human life (to drink, to use as radiation shielding, and to breath when cracked into its component hydrogen and oxygen), it can be used as a medium of energy storage (fuel cells) and it is the most powerful chemical rocket propellant known. Finding and using a water-rich NEO would create a logistics depot of immense value.
A key advantage of asteroids for resources is a drawback as an operational environment – they have extremely low surface gravity. Getting into and out of the Moon’s gravity well requires a change in velocity of about 2380 m/s (both ways); to do the same for a typical asteroid requires only a few meters per second. This means that a payload launched from an asteroid rather than the Moon saves almost 5 km/s in delta-v, a substantial amount of energy. So from the perspective of energy, the asteroids beat the Moon as a source of materials.
There are, however, some difficulties in mining and using asteroidal material as compared to lunar resources. First is the nature of the feedstock or “ore.” We have recently found that water at the poles of the Moon is not only present in enormous quantity (tens of billions of tons) but is also in a form that can be easily used – ice. Ice can be converted into a liquid for further processing at minimal energy cost; if the icy regolith from the poles is heated to above 0° C, the ice will melt and water can be collected and stored. The water in carbonaceous chondrites is chemically bound within mineral structures. Significant amounts of energy are required to break these chemical bonds to free the water, at least 2-3 orders of magnitude more energy, depending on the specific mineral phase being processed. So extracting water from an asteroid, present in quantities of a few percent to maybe a couple of tens of percent, requires significant energy; water-ice at the poles of the Moon is present in greater abundance (up to 100% in certain polar craters) and is already in an easy-to-process and use form.
The processing of natural materials to extract water has many detailed steps, from the acquisition of the feedstock to moving the material through the processing stream to collection and storage of the derived product. At each stage, we typically separate one component from another; gravity serves this purpose in most industrial processing. One difficulty in asteroid resource processing will be to either devise techniques that do not require gravity (including related phenomena, such as thermal convection) or to create an artificial gravity field to ensure that things move in the right directions. Either approach complicates the resource extraction process.
The large distance from the Earth and poor accessibility of asteroids versus the Moon, works against resource extraction and processing. Human visits to NEOs will be of short duration and because radio time-lags to asteroids are on the order of minutes, direct remote control of processing will not be possible. Robotic systems for asteroid mining must be designed to have a large degree of autonomy. This may become possible but presently we do not have enough information on the nature of asteroidal feedstock to either design or even envision the use of such robotic equipment. Moreover, even if we did fully understand the nature of the deposit, mining and processing are highly interactive activities on Earth and will be so in space. The slightest anomaly or miscalculation can cause the entire processing stream to break down and in remote operations, it will be difficult to diagnose and correct the problem and re-start it.
The accessibility issue also cuts against asteroidal resources. We cannot go to a given asteroid at will; launch windows open for very short periods and are closed most of the time. This affects not only our access to the asteroid but also shortens the time periods when we may depart the object to return our products to near-Earth space. In contrast, we can go to and from the Moon at any time and its proximity means that nearly instantaneous remote control and response are possible. The difficulties of remote control for asteroid activities have led some to suggest that we devise a way to “tow” the body into Earth orbit, where it may be disaggregated and processed at our leisure. I shudder to think about being assigned to write the environmental impact (if you’ll pardon the expression) statement for that activity.
So where does that leave us in relation to space resource access and utilization? Asteroid resource utilization has potential but given today’s technology levels, uncertain prospects for success. Asteroids are hard to get to, have short visit times for round-trips, difficult work environments, and uncertain product yields. Asteroids do have low gravity going for them. In contrast, the Moon is close and has the materials we want in the form we need it. The Moon is easily accessible at any time and is amenable to remote operations controlled from Earth in near-real time. My perspective is that it makes the most sense to go to the Moon first and learn the techniques, difficulties and technology for planetary resource utilization by manufacturing propellant from lunar water. Nearly every step of this activity – from prospecting, processing and harvesting – will teach us how to mine and process materials from future destinations, both minor and planetary sized-bodies. Resource utilization has commonality of techniques and equipment, the requirement to move and work with particulate materials, and the ability to purify and store the products. Learning how to access and process resources on the Moon is a general skill that transfers to any future space destination.
There was a reason that the Moon was made our first destination in the original Vision for Space Exploration. It’s close, it’s interesting, and it’s useful. Establishing a foothold on the Moon opens up cislunar space to routine access and development. It will teach us the skills of a space faring people. It makes sense to go there first and create a permanent space transportation system. Once we have that, we get everything else.
Destination: Moon or Asteroid?
Part I: Operational Considerations
Part II: Scientific Considerations
e to sail through the air at dizzy heights with ease? They are doing so in flying machines. Well, now that these supposed to be impossible feats have been accomplished, why be skeptical about anything?
The San Francisco Call included Felts’ design for the railway cars:
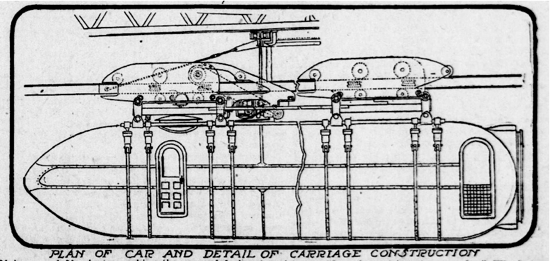
"Plan of car and detail of carriage construction."
Felts explained the design of his “bullet car” and the aerial railway:
“The car, which is bullet shaped, lays in surcingles which terminate in springs and plungers. The operator sits in a conning tower in the top of the car. Levers run back over his head to the engine. In place of the trucks beneath the car there are steel carriages above. These steel carriages will encircle the eyebeam tracks and all the wheels and rollers will be slotted at their tops, wide enough only to permit the passing of the drop arms. Should the wheels and rollers be removed by any cause, the carriages would clutch the track, preventing the car from falling, or, if any portion of the track should be taken away and the cars running at full speed the front carriage only would leave the track, causing the rear carriage to clutch the track before travelling the length of the car. This would prevent the car from plunging into space.
Felts clearly had a bigger vision for his railway system than just Oakland to San Francisco, explaining that a trip from Los Angeles to San Francisco could take just under four hours:
“My suspended auto motor railway, at the rate of 100 miles per hour, would make the same distance of 471 miles in 5 hours, including five stops of five minutes each,” said Felts. “This distance between San Francisco and Los Angeles could be shortened to 400 miles with the suspended auto motor railway, and the speed easily increased to 150 miles per hour, making the time between San Francisco and Los Angeles 3 hours and 39 minutes. The stops would be San Jose, Paso Robles, San Luis Obispo, Santa Barbara and Los Angeles.
High speed rail has been a hot button political topic in California, with the California High-Speed Rail Authority claiming that such a system today could link Los Angeles and San Francisco with trains taking just 2 hours and 40 minutes. California voters approved $9.95 billion in funding for high speed rail in 2008 but the project has many hurdles before it becomes a reality.