NATIONAL MUSEUM OF NATURAL HISTORY
Here’s How Scientists Reconstruct Earth’s Past Climates
Scientists apply different methods to the geologic record with the goal of better understanding and quantifying ancient Earth’s temperatures.
/https://tf-cmsv2-smithsonianmag-media.s3.amazonaws.com/blogging/featured/Haeckel_Thalamphora-002--saved-for-web.jpg)
Paleontologists have long recognized the power of using fossils preserved in the rock record to reconstruct the Earth’s past environments and climates. Today, animals and plants are associated with specific environments or climates - cactuses grow in dry deserts while polar bears live in cold northern latitudes. These same associations in the past provide a general understanding of how Earth’s climate has changed over the past 542 million years (the duration of the fossil record for complex life).
For example, the discovery of fossil palm tree stumps in Alaska and the unearthing of fossil alligator remains in the Arctic Circle suggested that the high latitudes were a tropical landscape 52 million years ago. Curator Scott Wing and postdoctoral fellow Richard Barclay with colleagues at the Smithsonian’s National Museum of Natural History have looked at how plant communities and plant traits track long-term changes in the climate. Fieldwork in now-arid Namibia has uncovered dropstones—large chunks of rock frozen to the underside of icebergs that are dropped into the ocean as the ice moves and melts. These dropstones were found in 650-million-year-old rocks, providing evidence that glacial conditions extended down to the equator at this time. But how do scientists actually take the temperature of ancient Earth from the geologic record?
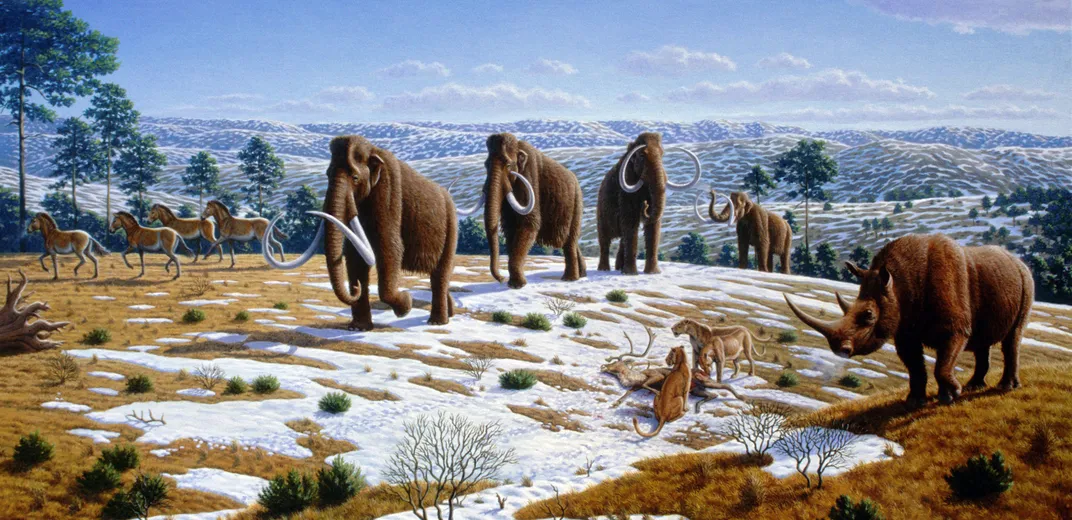
Although fossil communities and geological indicators like dropstones tell us if times were relatively warm or cool, they are coarse interpretations of the environment. Constructing a more finely detailed temperature curve is by no means a straightforward task. In our daily lives, we measure the outside air with tools like infrared probes or mercury-filled glass tubes to determine temperature in absolute ways using units such as degrees. Once we agree on a scale and understand how our tools reflect changes in temperature, anyone measuring temperatures along the same scale will know just how hot or cold something is. Our records of temperature using these modern tools go back hundreds of years. However, to look back even further in time, before humans were recording the temperature as they experienced it outside, we have to study the geologic record.
One way to measure past temperatures is to study ice cores. Whenever snow falls, small bubbles filled with atmospheric gases get trapped within it. In some places, so much snow falls that the older layers become buried and compressed into ice, locking away air bubbles in ice sheets and glaciers. With extremely careful drilling, we can extract long ice cores from these features to study the thousands of layers ice representing separate snowfalls and their trapped air bubbles. In controlled laboratory environments, we can measure the chemical makeup of the air that has been trapped - how much oxygen, carbon dioxide, and nitrogen gas was present in the atmosphere at the time it was buried in the ice. From these measurements, we can calculate past temperatures using empirical data on how these gases hold heat in the modern atmosphere. The temperature record recovered from ice cores goes back hundreds of thousands of years from glaciers that have persisted on landmasses like Greenland and Antarctica. However, ice sheets come and go and the oldest glacier is no older than a few million years. To get even older temperatures, we can’t just rely on what we can learn from ice.
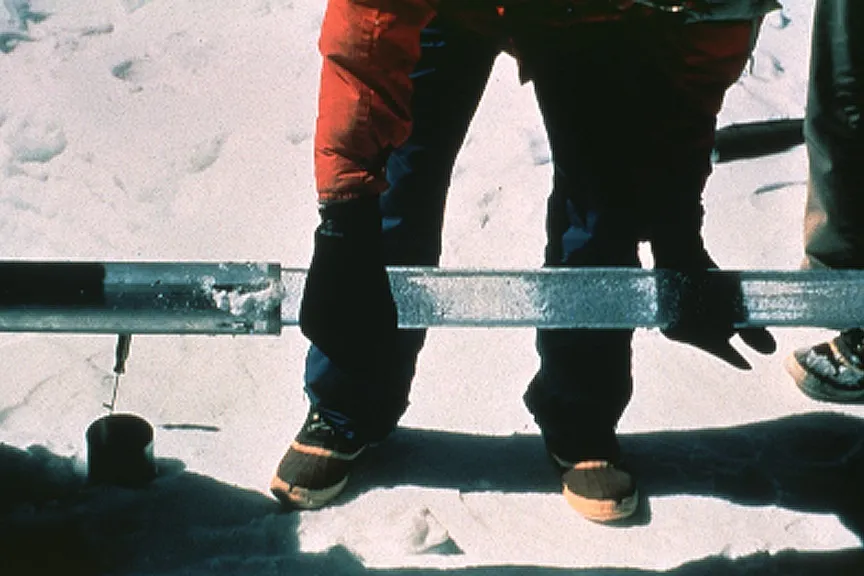
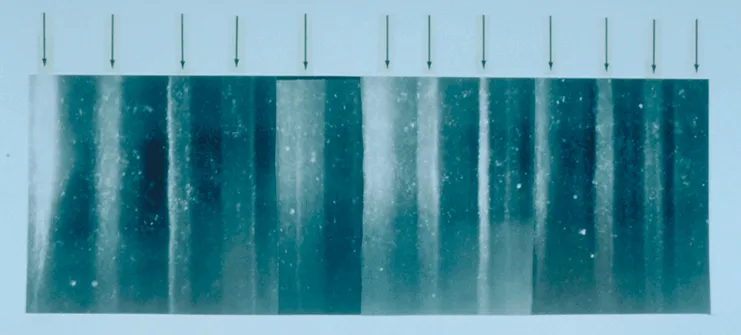
The most common method for measuring temperatures of ancient Earth uses naturally occurring isotopes. Isotopes are atoms of the same element that are heavier or lighter depending on how many neutrons are in its nucleus. Even molecules of water, composed of one hydrogen atom and two oxygen atoms, can have different weights depending on what isotopes of hydrogen and oxygen are bonded together. The two most common isotopes of oxygen in nature are oxygen-16 (8 neutrons) and oxygen-18 (10 neutrons). When the Earth cools down, the lighter, oxygen-16 found in seawater is locked away in the ice of high latitude glaciers due to evaporative processes, leaving behind relatively more oxygen-18 in the oceans. During warm global climates, melted ice returns oxygen-16-rich waters to the oceans. So the proportion of oxygen-18 to oxygen-16 in the ocean reflects the Earth’s climate even if we can’t see the ice. Earth Scientists recognize this oxygen isotope pattern between glaciated and ice-free climates, referring to it as the “ice volume effect”, and have since used it to reconstruct ancient Earth climates.
Oxygen isotope records are also preserved in the shells of marine organisms and the proportion of oxygen-16 and oxygen-18 can be revealed by analyzing the chemistry of pristine fossils. The fossils of larger organisms like corals or clams can be especially informative for revealing annual and seasonal temperature variations because these marine animals live for multiple years secreting season growth bands in a similar fashion to tree rings. However, well-preserved clams and corals can be geographically constrained, occurring only in the tropics or in near-shore environments. The true heavyweights for reconstructing ancient seawater temperatures are the microorganisms. Foraminifera, single-celled organisms roughly the size of the period ending this sentence, occur nearly everywhere in the oceans and have an exquisite fossil record. Thus, the fossil shells of foraminifera can provide a more complete understanding of the ancient ocean’s conditions across all latitudes and at most water depths. By measuring the oxygen isotope ratio in foraminifera, scientists like Brian Huber, a curator at the Smithsonian’s National Museum of Natural History, can reconstruct ocean temperatures more than 100 million years ago. Although they can fill in the gaps where macroorganisms are not preserved, foraminifera are unable to provide seasonal temperature records, highlighting the value of scientists working together on different aspects of the fossil record to build coherent records of the ancient climate.
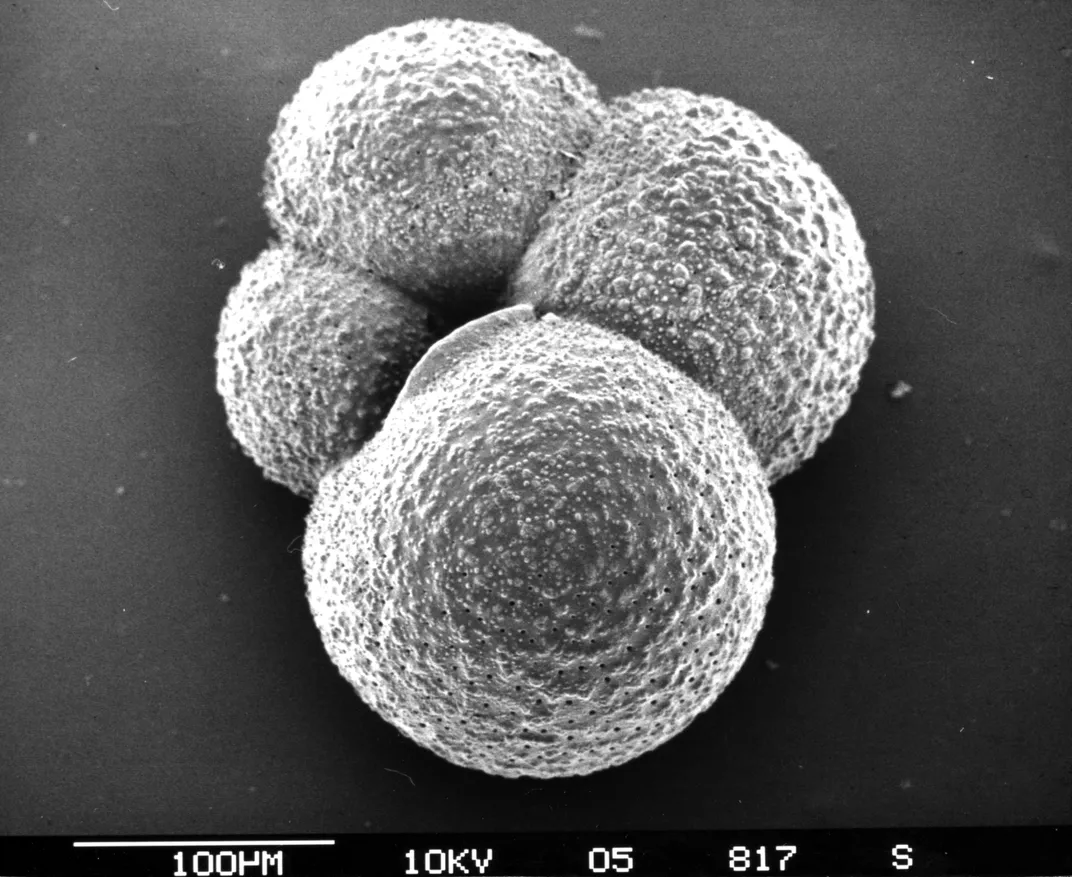
Thermometers, ice cores, isotopes. Because each of these approaches measures slightly different things - atmosphere versus ocean, gases versus isotopes, temperatures hundreds of years ago versus temperatures millions of years ago, seasonal versus annual versus time-averaged temperatures - matching them up with one another and building one continuous record of temperature through Earth’s history is a difficult task. This problem becomes even harder as we move further back in time because our ability to assign an exact age to a measurement decreases. To ensure we make the most accurate measurements possible, each proxy requires a specific expertise in the field and in the lab in addition to an understanding of how these methods work in modern settings through empirical observations and/or experimentation. Because these proxies tell us slightly different stories, scientists applying different methods to the geologic record with the same goal of better understanding and quantifying ancient Earth’s temperatures must collaborate to tell the complete story of our planet’s past. Events like the Paleoclimate Symposium at the Smithsonian's National Museum of Natural History provide forums in which scientists can share their knowledge, discuss these problems, and reach a working consensus as a community.
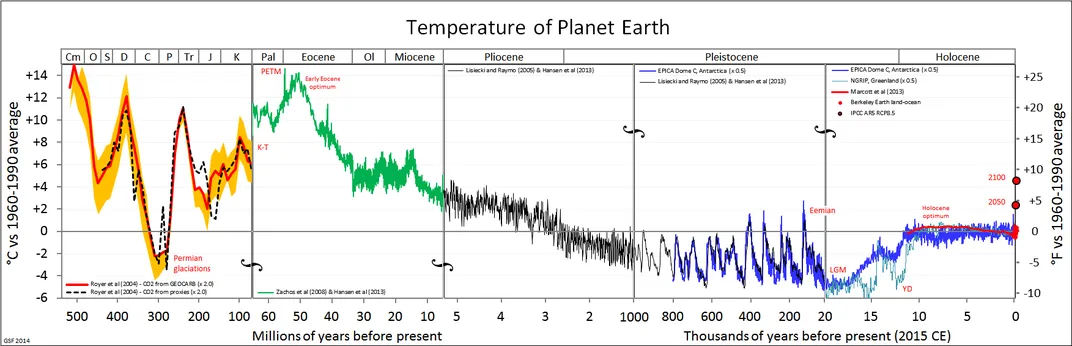