Forget Still Pictures. This New Observatory Will Make Astronomy Videos
The Universe in time-lapse.
:focal(3135x1555:3136x1556)/https://tf-cmsv2-smithsonianmag-media.s3.amazonaws.com/filer/a7/ad/a7adbc64-3ac0-44fb-89b8-7ce7a91fedcb/12b_am2020_sunset_live.jpg)
It will be the world’s largest digital camera. It will weigh in at three tons and be the size of an SUV. The telescope that surrounds it would cover a tennis court. Its 3.2 billion-pixel array will produce a new picture of the whole southern sky every three nights, with unprecedented detail, over and over, for years on end.
We’re accustomed to telescopes getting bigger and better, viewing ever fainter and more distant objects at increasingly higher magnification. The Hale Telescope on Palomar Mountain in California, the world’s largest mirror for much of the last century, begat Keck, twins that took over as largest with 10-meter mirrors in the 1990s. Keck begat the Large Binocular Telescope in Arizona, the current record holder with an 11.9-meter effective aperture. Designers of the next generation of telescopes strain themselves to come up with ever more over-the-top names. The Very Large Telescope. The Extremely Large Telescope. European astronomers once considered a concept called OWL, for Overwhelmingly Large Telescope.
The Vera C. Rubin Observatory will, in some ways, beat them all. J. Anthony Tyson, a professor of physics at the University of California, Davis, and chief scientist for the Legacy Survey of Space and Time, or LSST, which will be the observatory’s main job, has referred to it as “a form of celestial cinematography, the biggest movie ever.”
It’s been called a “discovery machine” and a new way of doing astronomy. Entire international conferences have been held to prepare scientists for LSST, knowing that few areas of astronomy will remain untouched by its findings. One example: Scientists expect it to discover 25 times the number of solar system objects now known to exist, from near-Earth asteroids to ice worlds beyond Pluto. And that’s just around one star.
The Rubin Observatory has been in the works for nearly three decades. In 2010, American astronomers, after thousands of hours of deliberation, named it their top priority for funding among large ground-based projects.
And it’s about to come online.
Tony Tyson was sitting in his office at Bell Labs in New Jersey one day in the early 1970s when a colleague (and future Nobel Prize winner) from down the hall walked in. George Smith, working with Willard Boyle, had recently invented a new type of electronic device. “He handed me a chip with a little gray spot measuring a couple of millimeters on a side,” Tyson recalls. “He said, ‘We’re calling it a charge-coupled device, and it can do images. In fact, we’re using it in the Picturephone.’ I said, ‘George, this is really great, but let me show you my plates.’ ”
Tyson, who trained as an experimental physicist but had developed an interest in astronomy, was taking images of galaxies the traditional way, on large glass plates. He was looking for evidence of gravitational lensing—distortions in the appearance of distant galaxies caused by the gravity of intervening galaxies or other objects.
“If you imaged enough galaxies, you could look for a telltale distortion,” he says. “But every glass plate was like a new detector. It was full of all sorts of chemical events. They called them ‘Kodak galaxies.’ It was a mess.”
While he continued to expose glass plates, Tyson started experimenting with CCDs. The early ones were “horrible,” he says, but they consistently produced the same “junk”—the same noise in the signal. Comparing images made it possible to remove the noise and produce deep, junk-free images of the universe.
As a result, Tyson became one of the first people to use CCDs to observe the heavens, as nearly every astronomer does today. Having been at the vanguard of one revolution, Tyson became the leading proponent of the giant survey telescope that’s now nearing completion atop Cerro Pachón, an 8,900-foot peak in Chile. First light for the Rubin Observatory is expected by the end of 2021, with full science operations to begin a year later.
“From cosmology to solar system science, we’ll see a lot of things change as a result of LSST,” says Federica Bianco, an astrophysicist at the University of Delaware and leader of the project’s scientific collaboration, which totals about 2,000 members from 23 countries. (Out of long habit, many scientists still refer to the whole project as LSST, although, officially, that’s just the sky survey. In January the observatory was named in honor of Vera Rubin, a pioneer of dark-matter astronomy. The telescope itself will be named for Charles Simonyi—software tycoon, space tourist, and donor, along with his wife, of funds for the project. Simonyi’s Microsoft colleague Bill Gates has also contributed.)
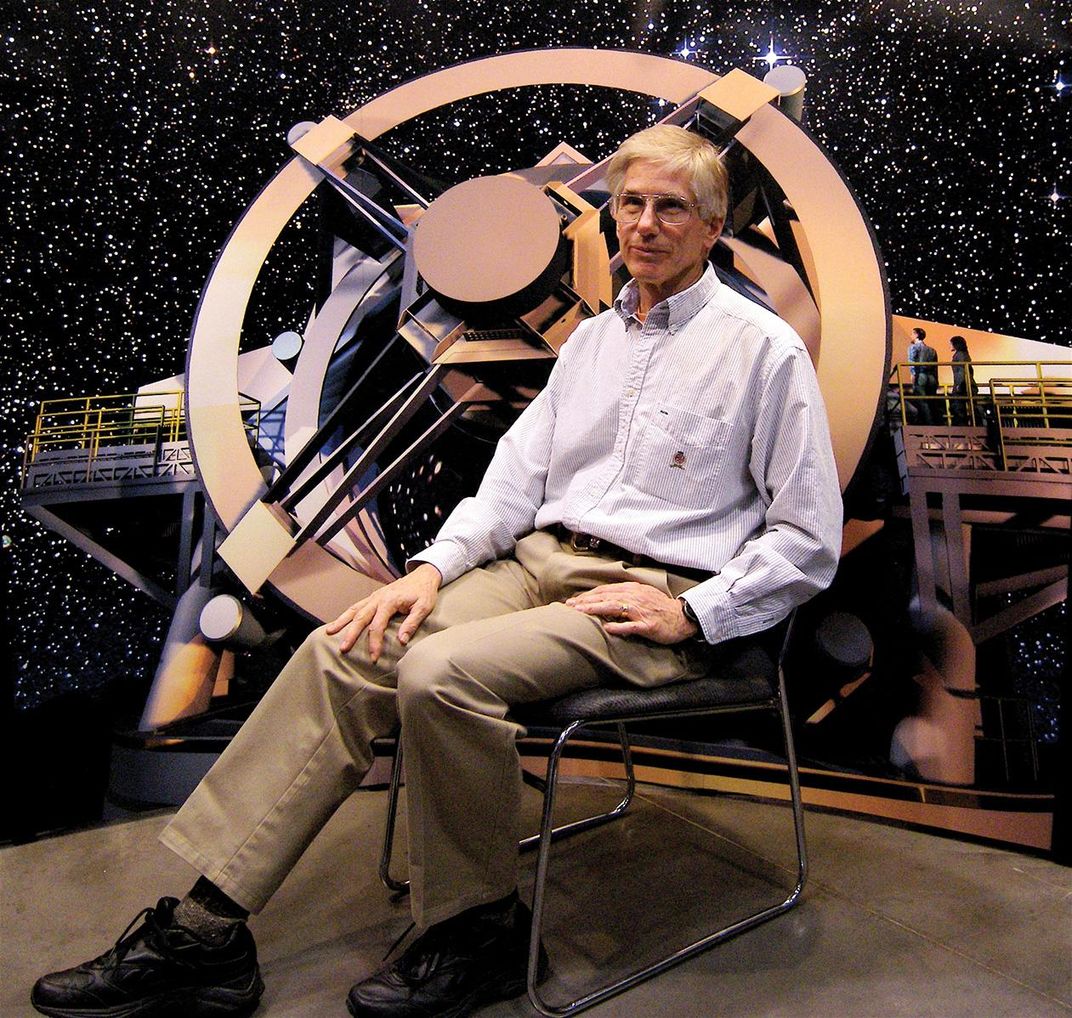
Over the course of the 10-year survey, the 8.4-meter telescope will scan the entire southern sky hundreds of times, allowing astronomers to map changes both near and far. That should yield a bounty of transient events—from exploding stars to asteroids in our own solar system that change position from night to night. “You can think of it as a 10-year movie of the universe,” says Rachel Mandelbaum, a physicist at Carnegie Mellon University and a leader of the group of astronomers who will use LSST to study dark energy.
Each change observed by the telescope will trigger an alert—perhaps 10 million notices per night—giving astronomers the chance to follow up on the discoveries with other telescopes. And every object LSST records—billions in all—will be logged in its database for study by astronomers around the world.
The Rubin Observatory’s mirror-and-camera combo will operate differently from other big astronomical telescopes. Most are designed to stare at a very small patch of sky for hours or even days at a time. These long exposures allow light from extremely faint objects (which usually means far away) to be recorded by cameras, spectrographs, and photometers. Their field of view, though, is tiny—astronomers often compare it to looking at the sky through a soda straw.
LSST, by comparison, has an enormous field of view—the equivalent of more than 40 full moons in area, or several thousand times what Hubble’s Wide Field Camera 3 sees. But it won’t linger on each patch of sky for long. It will take either a single 30-second image or a pair of 15-second images, which will be “stacked” together to provide deep views of objects as faint as 27th magnitude. (Dim Pluto has a greater magnitude of 14.) Then it will move on—in only five seconds—to the next field of view. On average, the survey will cover about a thousand fields each night—and the entire sky visible from its Chilean mountaintop in less than three nights. Then it will start the process all over again.
“The concept is very simple,” says LSST Director Steve Kahn, an X-ray astronomer who’s based at the project headquarters in Tucson. “But there are reasons it’s never been done before. It requires a novel optical design, a large array of sensors, physically large optics, and a large database management system. That’s a lot of technical challenges, most of which had never been encountered before. So LSST is more like an experiment than an observatory.”
The experiment began to take shape in the 1980s as Tyson developed larger and larger CCD cameras to probe dark matter—a so-far undetected form of matter that reveals its presence only by the gravitational pull it exerts on the visible matter around it. Measurements of the motions of stars in galaxies, and the cohesion of clusters of galaxies, suggest that there’s about six times more dark matter than normal matter in the universe.
Tyson was trying to map the distribution of dark matter by looking for images caused by gravitational lenses. In the 1990s, he and a colleague built the Big Throughput Camera (BTC), a 16 million-pixel array. They got time on a four-meter telescope in Chile in exchange for allowing other astronomers to use the BTC for their own projects. When two teams of astronomers used it to observe supernovas, their data led to the discovery of dark energy, a mysterious force that’s causing the universe to expand faster as it ages.
“We thought we could do even better than [BTC],” says Tyson, so he proposed a dedicated telescope-camera combination—originally called the Dark Matter Telescope—at a meeting in 1992. The idea gained steady support among astronomers until its 2010 ranking as their most desired large ground-based project. Federal funding followed, and LSST got a full “go” in 2014.
In terms of size, this 8.4-meter telescope won’t reign among the top five worldwide, but it makes up for that in speed. The CCD array has to read out its data fast enough for the camera to record faint objects even with LSST’s short exposure times. Then the three-ton assembly has to slew quickly to the next target field.
Casting the mirrors has been one of the project’s key challenges. Roger Angel, director of the mirror laboratory at the University of Arizona and a key figure in developing LSST, proposed a novel design. It uses three mirrors instead of the two found in most large telescopes, with two of them sharing the same piece of glass (see illustration, p. 28). “That had never been done before,” says Kahn.
The telescope was designed to fit the CCD camera, not the other way around. “What’s special about LSST is that it’s one integrated observatory—one end-to-end integrated system,” says Steven Ritz, LSST’s camera scientist and a professor of physics at the University of California, Santa Cruz.
After double-majoring in physics and music as an undergraduate, Ritz had gone on to get his Ph.D. in particle physics. Early in his career he worked at the European CERN laboratory and other facilities on such problems as identifying possible dark matter particles. He then decided to approach the problem from the astrophysics side, eventually becoming project scientist for Fermi, a space-based gamma-ray telescope.
“Fermi and LSST are both game changers in how they do this kind of science,” Ritz says. “They cover the whole sky many, many times. And they both provide one data set for answering questions about a broad array of topics.”
The LSST camera consists of three glass lenses that are among the most precise ever made, Ritz says. They will guide the image from the third (tertiary) telescope mirror onto an array of 189 16-megapixel detectors—“189 little cameras,” says Ritz. The survey will produce about 20 terabytes of data per night—a volume seldom seen outside big commercial data operations, says William O’Mullane, LSST’s data management director. “We look like a Google data center to some extent,” he says.
O’Mullane previously worked on the data processing requirements for two space telescopes, Hipparcos and Gaia, which have made the most accurate and detailed three-dimensional maps of stars in our region of the galaxy. He joined LSST in 2017, “happy to be building the next big thing.”
No image processing will be done at the observatory itself—the data will be sent immediately to the United States. Computers there will compare LSST images to previous views of each field and send out instant alerts when they find differences between the two. The entire process takes about one minute for each observed field.
Every object observed by LSST will be cataloged in a database that over time will swell to 15 petabytes (15 million gigabytes). The data management team, which numbers roughly 100 members, expects that machine learning and other tools will help users sift through the data. “We still have some work to do, but we have some time to do it,” says O’Mullane.
/https://tf-cmsv2-smithsonianmag-media.s3.amazonaws.com/filer/af/3f/af3fd165-4d60-4117-bd8a-0dae709e7c9a/12a_am2020_8593992554_c07735bf36_o_live.jpg)
The list of transient events includes stars that eclipse each other, stars that dim slightly when planets pass in front of them, and stars that puff in and out like beating hearts. Notably, it also includes comets and asteroids in the solar system. Tracking them could reveal if any are on a potential collision course with Earth. LSST is expected to add significantly to the inventory of known objects larger than 140 meters—a size threshold set by Congress for planning “planetary defense” measures.
Also of great interest to astronomers are the exploding stars known as supernovas. For a few days or weeks, such a titanic blast can outshine much closer stars, becoming bright enough to see from across the universe. Some supernovas result from the death of a massive star, while others—classed as Type 1a—are due to the destruction of a small, dead stellar core known as a white dwarf. Astronomers are still trying to understand the exact processes that power both varieties.
“When you see one or a few of these things, you understand them as novelties,” says Federica Bianco. “In the past, you would see maybe one supernova a century and it would be something amazing that would make you wonder if the Apocalypse was imminent. Today, the rate is about 10 per night. But LSST is expected to see a thousand supernovas per night.”
Bianco, who has participated in several smaller surveys of the night sky, has fought several bouts as a professional boxer (she has a 4-1 record with two knockouts). “It’s very primal but very simple,” she says of boxing. “Success is in the details, the ability to stay calm when the situation around you looks chaotic.” In addition to leading the sometimes chaotic LSST science collaboration, she also leads the sub-group devoted to transient phenomena.
Cataloging supernovas is an important tool for learning the nature and distribution of dark energy. LSST will see Type 1a supernovas in galaxies billions of light-years away, which means astronomers will be viewing them at different times in the evolution of the universe. These stars all happen to explode with the same characteristic brightness, so that measuring their apparent brightness is a handy way to determine their distance. And knowing their distance reveals how fast the universe was expanding—due to the pull of dark energy—during different epochs in the past.
“The measurements are all challenging,” says Rachel Mandelbaum, who has spent her career observing the universe on the largest scales. “But it’s valuable to have multiple ways to get the expansion of the universe and the distribution of dark matter.”
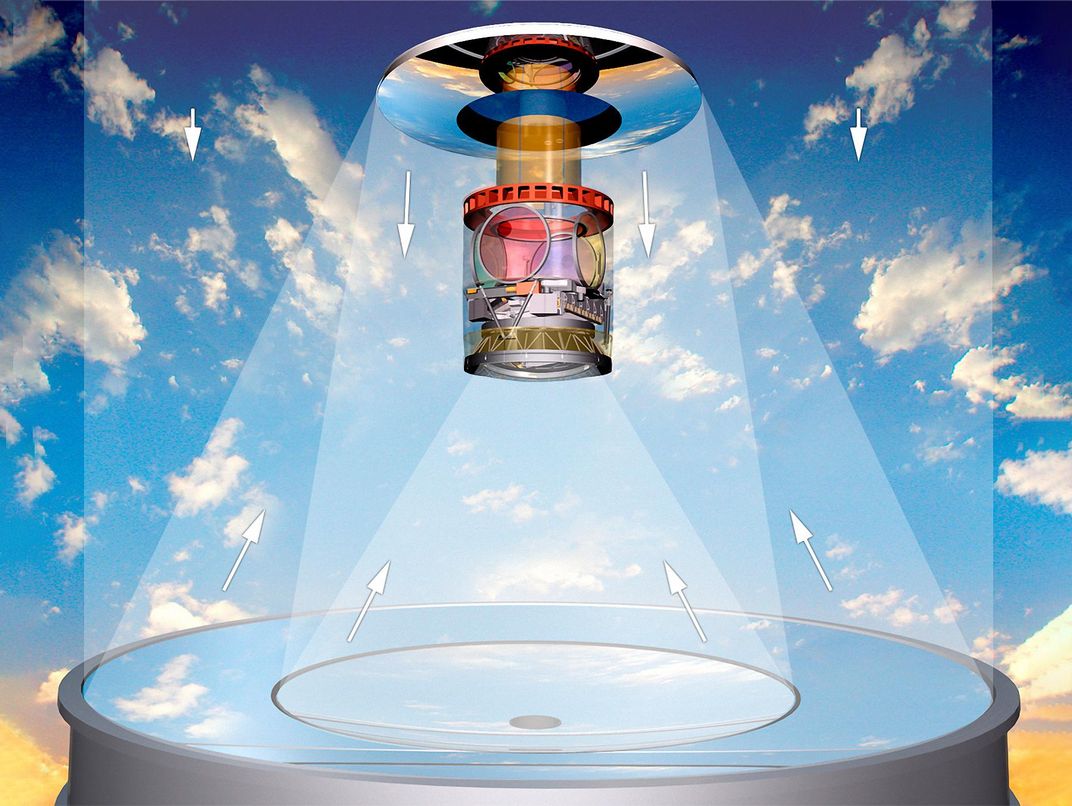
Another technique uses a phenomenon known as weak gravitational lensing, caused by light passing through clouds and filaments of dark matter. The effect is subtle, however, so it’s almost impossible to see. Astronomers need to view many galaxies (LSST should map 10 billion of them, “more galaxies than there are people on Earth,” Kahn says) and use statistical techniques to tease out what’s really happening. With enough observations at varying distances, they hope to map the “clumpiness” of matter, including dark matter, intervening between Earth and remote galaxies.
That, in turn, provides insight on “how cosmic structure has grown over time,” says Mandelbaum. Weak lensing and other techniques have shown that dark matter congregates in long filaments, forming a “cosmic web.” Clouds of hydrogen and other elements are pulled in by this dark-matter web. Where strands of the web intersect, the clouds have collapsed to give birth to galaxies.
LSST should provide measurements of the cosmic structure over the last nine billion years or so, which is far longer than measurements made by previous weak-lensing surveys. The technique will complement LSST’s supernova observations, Mandelbaum says. “When you put those two together, it’s quite powerful.”
These are just some of the things astronomers expect from their new observatory after all these years of planning: from potential Earth-crossing asteroids to universe-size structure. And these are things astronomers know to look for. “When you take out the known things and look at what’s left over,” says Kahn, “we might have a hundred thousand objects a night.” That’s big.