Would We Know Alien Life If We Saw It?
And have we already seen it on Mars?
/https://tf-cmsv2-smithsonianmag-media.s3.amazonaws.com/filer/4d/5a/4d5a5f45-802a-4310-8363-d509ad08aab1/14a_am2016_arrestingmarsasol744b_p2351_l257f-b744r1_live-web-resize.jpg)
At this moment, seven robotic spacecraft are roving or orbiting Mars, taking photos, gathering data, and generally doing the bidding of scientists back on Earth. After 15 years of this continuous robotic presence, we know the Red Planet better than any world besides our own. And planetary scientists have an answer, finally, to one of their oldest and most fundamental questions: Could Mars support life?
The answer is yes: certainly in the past, and very possibly today. In 2013, less than a year after Curiosity touched down in the ancient lakebed Gale Crater, John Grotzinger, the project’s principal investigator, announced with confidence: “We have found a habitable environment,” one where substantial amounts of surface water existed billions of years ago. What’s more, the Curiosity science team is convinced that the lakes and streams lasted for long periods, perhaps millions of years.
Another announcement, just as momentous, followed last September: Water still flows on Mars today—at or very near the surface. For more than a decade, NASA’s strategy in exploring Mars has been to “follow the water”; the agency reasons that wherever there’s water, we might find life. Now, having made the case for water, space agencies are preparing to launch Mars missions whose primary purpose is to search for evidence of biology. And, unlike earlier searches, these missions have a real chance for success.
In the 1960s, the first generation of planetary scientists tried to come up with a single suite of instruments (for what became the 1976 Viking landers) that could settle definitively whether life exists on Mars. Ultimately, they failed. Scientists now suspect that past experiments in Martian biology asked questions that were too narrow or even wrong.
“Defining life is a problem,” explains Carol Cleland, a University of Colorado philosopher who has spent more than a decade examining the scientific and philosophical literature on the nature of life. “If your definition is wrong, you’ll look for the wrong thing—and be liable to miss all kinds of weird forms of life. Even today, we haven’t gotten away from an Aristotelian definition.”
More than 2,000 years ago, Aristotle defined living beings as those that metabolize (consume nutrients and eliminate waste) and sexually reproduce. That definition served well enough until the middle of the 20th century, when scientists learned about DNA and came to understand that the predominant life-form on Earth is the single-cell organism. (Indeed, complex multicellular life doesn’t appear in the fossil record until less than a billion years ago.)
Many single-cell creatures defy Aristotelian ideas about metabolism and reproduction. Some don’t consume organic nutrients at all. A bizarre marine microbe called Shewanella, for example, gets its metabolic energy by using “nanowires” that draw electrons directly from rocks. Some organisms don’t need sex to reproduce: They “fragment” directly from the parent. Still others act as if they’re alive at some times, dead at others. Viruses, for example, can lie dormant for centuries in a crystalline state.
In the past few decades, scientists have found many “extremophiles,” which survive quite nicely in environments once thought to be lethal: in superheated geysers, on the bottoms of Antarctic glaciers, in the crushing blackness of the deep ocean.
If terrestrial life has turned out to be far stranger and more adaptable than we once thought, how much weirder could it be in an alien biosphere like Mars?
Yet there’s reason to hope we’ll find familiar organisms too. “The argument for water-based and carbon-based life is never stronger than on Mars,” says David Des Marais, principal investigator of space science and astrobiology at NASA’s Ames Research Center in California. “Some folks like to speculate that solvents other than water might also support life,” he notes. “While one can never absolutely deny the possibility of ‘weird life’ based on an alternative solvent, water is particularly favorable for Mars because the environment of Earth has been more similar to that of Mars than that of any other planet in our solar system.”
Since we have to start somewhere, Des Marais and others argue that we should look for familiar forms of life first; we can worry about the life-forms we don’t know later. “Pick your best shot” for success, he says.
Haven’t we done this before?
On July 20, 1976—the seventh anniversary of the Apollo 11 moon landing—NASA’s Viking 1 set down near the equator of Mars, the first fully successful mission to the planet’s surface. Six weeks later, its twin, Viking 2, landed, a bit farther north, on the opposite side of Mars. Panoramic images from the two stationary spacecraft (there were no wheeled rovers on this first expedition) confirmed a pebble-strewn, desert landscape devoid of any obvious signs of life.
Each Viking was equipped with a scoop for digging shallow trenches in the Martian “soil” (actually sandy regolith bombarded by ultraviolet radiation; it bears little resemblance to terrestrial topsoil) to obtain samples for three experiments inside the spacecraft that were designed to look for evidence of biological activity. A gas exchange experiment fed nutrients and water to the soil samples and looked for signs that organisms either consumed or released one of the nutrients. A pyrolytic release experiment exposed soil to light and a synthetic Martian atmosphere tagged with radioactive carbon-14, then removed the atmosphere and cooked the sample to release gases that were examined for evidence of biomass containing carbon-14: a proxy for photosynthesis. Astrobiologists had their highest hopes for the labeled-release experiment: Soil samples were fed organic nutrients tagged with carbon-14, and the air around the sample was monitored for radioactive carbon dioxide, which would have been exhaled by metabolizing microorganisms.
The results frustrated everyone. The gas exchange experiment was negative for microbes but suggested that the soil has highly reactive chemical compounds. In the pyrolytic release experiment, one sample was positive, but so was a control sample that had been sterilized, suggesting that something other than biology was at work. The labeled-release experiment on both spacecraft detected carbon dioxide at first, but not again when retried a week or two later. And the clincher: a non-biological experiment—a gas chromatograph–mass spectrometer (GCMS)—saw no trace of organic materials in the Martian regolith. This was a surprise, since organic molecules are common in meteorites, including rocks found on Earth that originated on Mars. And the apparent lack of organic matter seemed to rule out any positive results from the biology experiments. Considering all these results together, the Viking science team issued its disappointing verdict: no life at either landing site.
Did the Viking experiments work right? Was the GCMS broken? Did harsh solar ultraviolet radiation (Mars has no protective ozone layer) or some unknown chemical such as a strong alkaline oxidizer (think bleach) destroy all organic molecules on the Martian surface? Or was the design of the three biological experiments too rooted in terrestrial assumptions, and the Earth-type nutrients and water poisoned or drowned Martian organisms adapted to a hyper-arid and otherwise un-Earth-like environment?
For 40 years the ambiguous Viking results have fueled scientific debate. Gilbert Levin, principal investigator for the labeled-release experiment, is convinced to this day that Vikings 1 and 2 found evidence of life on Mars. NASA’s Phoenix spacecraft, which landed near the planet’s north pole in 2008, re-started the argument when it confirmed that the chemistry of the Martian soil may in fact destroy organic material—which could explain at least some of the Viking findings.
The main culprit seems to be perchlorate salts, a highly reactive oxide of chlorine found at the Phoenix landing site. At the low temperatures prevalent on Mars, perchlorates would not themselves react with organic matter, but the planet’s harsh radiation could split them into more reactive compounds. In 2013 Richard Quinn at the Ames center conducted experiments in which perchlorates irradiated with gamma rays seemed to reproduce the puzzling findings of the labeled-release experiment.
Although perchlorates might destroy organic compounds exposed to radiation on the Martian surface, could microbial life exist protected within rocks or underground? In fact, last year Curiosity’s Sample Analysis at Mars (SAM) instrument discovered two types of complex organic molecules in powdered samples drilled from inside a mudstone at Gale Crater. One molecule even resembled a fatty acid found in the cell walls of terrestrial organisms. Although the Curiosity scientists made no claims about Martian life, we now have proof that under certain circumstances, organic molecules can survive on the planet.
Where to go, how to look
Whether the search is for current life or for fossil evidence of past life, “follow the water” continues to be a useful strategy. Fortunately, in the 40 years since Viking, scientists have found abundant evidence of water. Data collected from orbit and from the Spirit, Opportunity, and Curiosity rovers suggest that the planet once had an ocean with a volume greater than Earth’s Arctic Ocean. And the water likely shifted over time. Mars’ axial tilt—astronomers use the term “obliquity”—is wildly variable, and the large historical swings in obliquity, which occur on time scales of hundreds of thousands or perhaps a million years, “could also lead to global redistributions of water,” says Dirk Schulze-Makuch, a professor of astrobiology at Washington State University. Redistribution may explain surface features that look to have been carved by running water within the past million years, long after the large ocean disappeared.
Imagery taken from orbit, going back to Viking, has shown morning fog and mist rising from the floor of Martian canyons, leading scientists to theorize that liquid water may still be trapped under the surface. (Schulze-Makuch even speculates that Martian organisms might draw water directly from the atmosphere.) And last September, high-resolution images from the Mars Reconnaissance Orbiter revealed that even today, water—actually, brine that can stay liquid at cold temperatures—flows down steep slopes in the Martian spring and summer.
The discovery that liquid water has persisted on the surface of Mars over long periods gives hope that life arose there, and that it found a way to adapt to harsh conditions, which changed as the surface water disappeared. “Life is a kind of planetary pest,” says Schulze-Makuch. “An infestation, once started, is very difficult to get rid of.” Invoking Carl Sagan’s famous dictum that extraordinary claims require extraordinary evidence, he adds, “I think the extraordinary claim is that Mars always was sterile.”
Within five years, NASA and the European Space Agency hope to test that optimistic idea. The next major mission to the surface of the Red Planet is ExoMars 2018, a joint project of the European Space Agency and the Russian space agency Roscosmos, which is supplying the Proton rocket. Currently slated to soft-land on Mars in January 2019 (or two years later if the launch date slips, as has been rumored), ExoMars will deploy a rover equipped with a drill capable of boring down six feet. The goal, explains Mark Sims, a professor of astrobiology and space instrumentation at the University of Leicester in England, is to obtain samples from depths at which they’ve mostly been shielded from intense radiation that would break organic molecules apart.
In choosing a landing site for ExoMars 2018, project scientists used orbital data to scout out places with sedimentary rocks, especially fine-grained clays, that clearly formed in the presence of water, as in an ancient lakebed. The ideal sample rock would be very ancient—four billion years old or so, buried most of that time, and only freshly exposed to Mars’ harsh surface environment by erosion or a relatively recent landslide. The ExoMars project narrowed the potential landing sites to four, the top candidate being Oxia Planum, a smooth, flat plain with only a light dust covering, so more of the surface rock should be exposed. Here, 18 degrees north of the Martian equator, the ExoMars rover will look for evidence of biology.
Finding visible fossils—say, the remains of bacteria like those seen in some ancient Australian rocks—would be wonderful, but for a number of reasons extremely unlikely. For one, such fossils would almost certainly be too small for the ExoMars close-up camera to resolve. So just as Viking did 40 years ago, the ExoMars search focuses on chemistry.
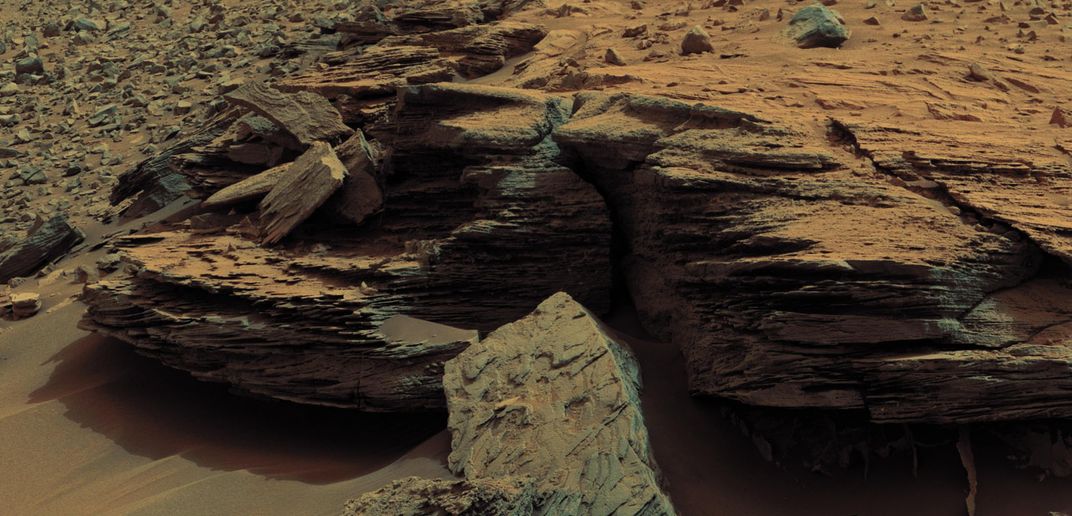
The rover’s main instrument for life detection is called MOMA, for Mars Organic Molecule Analyser. It will use two kinds of spectrometer to analyze drilled samples for traces of organic molecules, and scientists hope to be able to distinguish compounds associated with biology from those that are non-biological. The instrument will also analyze any organic compounds’ chirality, or “handedness.” Amino acids and other molecules exist in either right-handed or left-handed forms. All plant and animal life on Earth is based on left-handed amino acids (although some microbes can, in a pinch, consume the right-handed versions of nutrients). An ExoMars sample with a 50-50 mixture of both chiralities would imply geologic origin, whereas a predominance of one chirality over another would suggest a biological origin—that is, if Martian life also has a preferred handedness.
NASA plans to launch its fifth rover (a near copy of Curiosity) to Mars in July 2020. It will land seven months later and begin searching for rocks that can be sealed in a container and returned to Earth by a future spacecraft, still to be specified. Scientists have long hankered for a mission that can bring Mars rocks home, so they can analyze them on Earth with more sophisticated instruments than can fit on a lander. Mars 2020 is the first half of that mission, and it will be up to the 2020 rover to identify the precious few rocks that have the best chance of containing bio-signatures, or evidence of life.
The main life detection instrument on the 2020 rover is called SHERLOC, for Scanning Habitable Environments with Raman & Luminescence for Organics and Chemicals. Principal investigator Luther Beegle of NASA’s Jet Propulsion Laboratory describes it as “a stand-off instrument” mounted on the rover’s robotic arm. “We don’t want to touch samples and possibly detect life we brought with us to Mars,” he says. Instead, from two inches away, SHERLOC will shine far-ultraviolet lasers on rocks to cause their constituent chemicals to either scatter light or fluoresce (emit light). The resulting spectrum should reveal the chemical fingerprints of any organic molecules in the rocks. Promising samples would be candidates for caching—again while taking steps to avoid contamination—and eventual return to Earth.
The Mars 2020 team has yet to choose its landing site—eight candidates are in the running. Selecting the right location is critical, since the two-part mission is a multibillion-dollar investment. If no biology is found at the 2020 site, or if the answer is muddled, as with Viking, critics might say NASA wasted its money going to the wrong place.
That’s one of many potential pitfalls in the search for life on Mars. Because of budget constraints, not every proposed biology experiment can fly, so some worthy approaches to life detection will go untried. A “Life Marker Chip” originally picked for ExoMars 2018 would have used antibodies to detect organic molecules, similar to tests used in the medical world. But the payload was dropped along with several other instruments to save costs and reduce weight.
First, do no harm
Another constraint on scientists looking for Martian life: the “planetary protection” requirements. By international agreement, spacecraft landing in regions on Mars where water might exist have to be cleaned thoroughly before leaving Earth, for fear of exposing possible Martian organisms to terrestrial contamination or—equally bad from a science standpoint—raising doubts as to whether a “Martian” organism actually came from Earth. Cleaning large, complex spacecraft with dry heat is difficult and expensive. For now, Mars mission planners avoid landing sites that might have liquid water, even though those are the sites most likely to have life.
The team behind a proposed mission called Icebreaker, which would send a small, Phoenix-like lander to high Martian latitudes where liquid water might exist, is trying other approaches to removing microbial contamination, such as chemical cleaning of any equipment that comes in contact with the sample. Icebreaker (at this point an unfunded concept) would carry a drill capable of penetrating three feet into the soil. An onboard Signs of Life Detector (SOLiD) would analyze the drilled samples for bio-signatures: organic molecules, proteins, polysaccharides, and nucleic acids including DNA.
Only by canvassing for a wide range of possible bio-signatures can scientists hope to avoid philosopher Cleland’s terra-centric trap: looking only for the kind of biology we see on Earth. “There’s a difference between searching for life on Mars and searching for different life on Mars,” says Chris McKay, a senior scientist at the Ames center and the principal investigator for Icebreaker. “Martian life could be carbon-based and still be alien. The real hope is to find a second genesis: that is, evidence of life not related to the tree of life as it evolved on Earth.”
Just as rocks blasted off the Martian surface by ancient impacts have found their way to Antarctica, organic material from Earth may have already been transported to Mars, says McKay. If that’s true, any microbes found on Mars might be our long-lost distant cousins. Hence the need to test for Earth-like nucleic acids like DNA. Says McKay: “We need to define alien life not geographically—that is, being from another planet—but biochemically.”
Most biology on Earth is based on roughly 20 amino acids, but in nature there are some 500 such compounds. Discovering Martian life-forms based on different amino acids would point to a second genesis, independent of our own. So would finding Mars life that used the same amino acids as terrestrial organisms, but with right-handed chirality.
Cleland applauds any search that includes possible alien biochemistry. “We need to look for features all life would display, regardless of chemistry,” she says. “Life is a self-organizing system. So look for patterns or unexpected degrees of complexity.” Life, she continues, is “an out-of-equilibrium state. We need to investigate anomalies: stuff that shouldn’t be there.”
She is especially intrigued by repeated detections of methane gas on Mars, starting with Mariner 7 in 1969, again by Mars Express and Earth-based telescopes in the early 2000s, and most recently by Curiosity, which detected mysterious, short-lived burps of methane on the surface at Gale Crater. The methane shouldn’t be there; if it originated in the distant past, it would have dissipated in the Martian atmosphere in just a few hundred years. The detections suggest that somehow it’s being replenished.
The jury is still out as to whether the source is geological or biological. In a review article published last year in the Journal of Astrobiology & Outreach, Yuk Yung of Caltech and Pin Chen of the Jet Propulsion Laboratory give two hypotheses: the methane could be due to gas-water-rock chemistry or to microbes that exhale methane. “The former,” they write, “implies the existence of environs offering liquid water and chemical sources of energy—i.e. habitability—while the latter implies the discovery of life on Mars.”
The European Trace Gas Orbiter on an ExoMars mission to be launched this year (which also will drop off a small descent module to test landing technology for the 2018 mission) will collect data from orbit that should tell scientists more about Martian methane. But this one instrument is unlikely to settle the question of whether the methane comes from a biological source. In fact, write Yung and Chen, solving the puzzle will require a “research effort across many disciplines,” as well as “major technological advancements.”
While the prospect that living Martian organisms are exhaling methane right now is exciting, McKay cautions against being so intent on finding something alive on Mars that we overlook how significant it would be to stumble across evidence that something lived there in the past. “A dead rabbit on Mars would be powerful evidence of life on Mars,” he says. “So would the discovery of a single chlorophyll molecule—because although the molecule is not alive, you can’t get to chlorophyll without life.”
Perhaps the most persuasive evidence of past life would be recognizable fossils—hence the tremendous flurry of excitement in the 1990s when several scientists thought they might have discovered ancient micro-fossils of bacteria in the four-billion-year-old Martian meteorite ALH 84001, retrieved from the icy wastes of Antarctica. Most scientists now believe the claim is unfounded; most of the “evidence” can be explained non-biologically.
Even the identification of ancient micro-fossils on Earth is controversial. The “Apex chert,” discovered in Australia in the 1980s, originally was believed to contain the world’s oldest fossils, dating back 3.46 billion years. Almost 30 years later, more sophisticated instruments and reinterpretation of the local geology showed that the “fossils” are inanimate minerals, not the remnants of once-living bacteria.
The identification of life on Mars therefore is unlikely to rest on a single picture, or even a single piece of data. “There is no ideal bio-marker, unless life walks up to you and waves,” declares Mark Sims, the ExoMars team member who led development of the now-canceled Life Marker Chip. His worry is not just false positives—evidence that wrongly suggests life on Mars. He also worries about “evidence for Martian life successfully extracted from the Martian soil but lost en route to detection.” He and three colleagues wrote a paper in 2012 analyzing how various organic compounds can end up stuck to steel, titanium, and other materials commonly used in spacecraft instruments, and never even make it to the detector. If Sims sounds like a worrier, he has the scars to prove it. He was the mission manager for the British-built Beagle 2 lander, which landed on Mars in 2003 but never phoned home. He never got a chance even to begin that search.
Though finding life on Mars appears a near-impossible task, scientists have reason for optimism. Compared to our knowledge in the Viking era, we now know more about biology, including weird biology, and much more about Mars. Orbiting spacecraft are able to take high-resolution images and map the mineralogy of any site we choose to visit. In fact, NASA is so confident that extraterrestrial life is waiting to be discovered that last year the agency’s chief scientist, Ellen Stofan, said: “I think we’re going to have strong indications of life beyond Earth within a decade, and I think we’re going to have definitive evidence within 20 to 30 years.”
And what if we do?
“I don’t think the response should be to send Earth life to compete with it,” says McKay. “We’d need to take steps to protect the second genesis.” The planetary protection dilemma will only get worse as the spacecraft become more complicated. (And nobody yet knows how to do a human Mars expedition, which some consider our best bet for finding life, without compounding the risk of contamination.) In 1975, the Viking landers were assembled in clean rooms, then put into a giant oven and baked at 200 degrees Fahrenheit—what chefs would call a “cool” oven—for 30 hours. But prolonged heat would cause some modern electronics and seals to fail. Like McKay and his Icebreaker team, spacecraft engineers are hoping to come up with new cleaning methods.
“We also need to think seriously about the risk of bringing [Martian samples containing life] back to Earth, and observe isolation techniques comparable to what we do for Ebola,” McKay says. His fear is not that alien organisms would cause a plague on Earth, but that they could displace some terrestrial life-forms, just as introduced species, like kudzu, have in new environments on Earth.
The best reason to be hopeful about finding Martian life may be the odds. Biology is likely either to be confined to Earth, or to be everywhere. The late, great science fiction writer Isaac Asimov once observed that phenomena in the universe are either unique or universal: Two is an impossible number.