A Critical Test for NASA’s Monster Rocket
Facing immense challenges, the agency bulls ahead with its Space Launch System.
:focal(2625x2278:2626x2279)/https://tf-cmsv2-smithsonianmag-media.s3.amazonaws.com/filer/30/d1/30d17d89-22ba-4c90-b133-432e6cdf1c65/15l_fm2020_maf_20190626_p_loxstarollout-316_live.jpg)
From a test-stand catwalk almost 300 feet above the ground at NASA’s Stennis Space Center in southern Mississippi, Maury Vander and I take in a view of the 14,000-acre rocket propulsion test complex, its 200-square-mile acoustical buffer zone, and distant New Orleans. Vander, who started at Stennis in 1989 after graduating from the University of New Orleans with a degree in mechanical engineering, is the chief of test operations for the approaching Green Run, a long-awaited test that will certify for flight the core of a rocket that has been in development for a decade.
“I spent my first 23 years in the best job on this site, a test engineer,” he says. “We made the ground shake and the weather change.” That was during the space shuttle era. The test that Vander and his colleagues are preparing for now is for a monster so different from the shuttle that the test stand itself has undergone a six-year modification, including the addition of a million-pound steel framework to extend and strengthen the existing structure. This spring the stand will support most of the Space Launch System, the rocket that will push beyond Earth orbit for the first time since the Apollo program. Before those deep-space missions can lift off, the SLS must first pass a bolted-down trial of the rocket’s big core stage, the stack of tanks and main engines that forms its backbone. The trial is called a “Green Run” because some of the rocket hardware is new and has never been tested with all of the pieces running together, as they will at Stennis.
“I’ve been here 30 years now and [SLS] is the biggest project I’ve been involved in,” says Vander. “I watched the Apollo videos from the sixties, and I like to think in 40 or 50 years people will be watching our activities now and saying, ‘Wow, they did that?’ ”
The Space Launch System is a critical part of NASA’s next moonshot, the Artemis program, named for the sister of Apollo, which promises to send a woman and a man to the moon by 2024. With an upper stage and payload atop its core, the new moon rocket will stand 322 feet tall. That’s 41 feet shorter than Apollo’s Saturn V, but on the launchpad, the SLS will produce 15 percent more thrust—8.8 million pounds, as opposed to the Saturn’s 7.5 million pounds.
By a 2010 order of Congress, the SLS relies heavily on long-proven hardware from the space shuttle, including engines, solid-fuel boosters, fittings, and tank design. It also got a head start from pieces developed and tested for the earlier Constellation space exploration program, which, before it was canceled in 2010, had begun work on its own heavy-lift launch vehicle, the Ares I and V rockets. Because of this double legacy, there was an expectation outside NASA that SLS development would be, if not a breeze, not a reach either. Although SLS relies heavily on hardware from the reusable shuttle, it is a simpler machine because nothing from the SLS launch vehicle will return for refurbishment and reuse.
But it turns out that nothing about a heavy-lift launch vehicle is simple, especially one designed to provide the performance that the deep-space Artemis program requires. High performance requires invention; invention requires test, refinement, and more test; and lives are at stake. As far as NASA and its principal contractor Boeing are concerned, the SLS is a brand new beast, and its parts must prove themselves individually and collectively. If any of the technical challenges are not addressed adequately, the result could be what NASA managers call a “bad day”—multimillion-dollar damage on the ground or the catastrophic loss of a mission.
“Every day is a new challenge, and you can quote me,” said Chris Cianciola, Marshall Space Flight Center’s deputy program manager for the Space Launch System. Cianciola has shifted his workplace from Marshall in Huntsville, Alabama, to the Michoud Assembly Facility, the Louisiana complex 15 miles northeast of downtown New Orleans where the enormous pieces of the rocket core stage are being welded and bolted together. “I can’t tell you what you will see tomorrow,” he says. “What we’re doing on the core stage—each operation is the first time it’s being performed.”
I visited Michoud in October, while workers were assembling the core stage that will fly on the Artemis I launch. (On that mission, now planned for 2021, an uncrewed Orion spacecraft will swing around the moon, get a gravitational boost to an orbit 40,000 miles above the moon, then return to splash down three weeks after launch.) Technicians were installing the four main engines; afterward, they would check all the fuel and electrical connections. “There are still some feed lines left to install,” said Steve Ernst, Boeing’s final assembly engineering leader, as we walked along the core’s 212-foot length. “And we’ll make sure all the boxes are all talking to each other, checking sensors to get their feedback. That’ll take several weeks.”
We’re inside Michoud’s biggest enclosure, Building 103. It covers 43 acres. One could call 103 a single-story building, but that story has a ceiling more than 40 feet above the concrete floor. Among NASA facilities, the Vehicle Assembly Building at Kennedy has a claim on vertical grandeur, but Michoud rules in acreage.
Among the hardware that the SLS inherited from the space shuttle are its liquid-fuel main engines. Though long proven as star performers during the shuttle era, they present several challenges when taking their place on the SLS. One headache is how to finish off the engine installation without collateral damage. The engines themselves occupy so much of the limited space inside the fairing that they leave barely enough room around their bracing struts for the dense maze of wiring, actuators, controller boxes, tubing, four auxiliary power units, five helium tanks, and everything else needed to start and bring the engines to full power in seconds, then run them for more than eight minutes, and safely shut them down. Adding to the Twister-like challenge, the fairing permits technicians to reach their work only through a bottom hatch between the four engine nozzles or through panels on the side.
Some tasks have been simulated with digital mannequins to ensure humans can make all the necessary connections without leaning against, stepping on, or snagging something delicate. Avoiding damage during installation is a careful, heartfelt effort at NASA. Damaged Teflon wire insulation likely contributed to the fatal Apollo 1 capsule fire in 1967.
The engine section’s elbow-room problem arises from an early decision about the diameter of the core stage. SLS planners considered going big and copying the 33-foot-diameter of the first stage of the Saturn V, but that would have increased the rocket’s weight and cut back on payload. It would also have hiked equipment costs because Michoud’s handling equipment and processing rigs had already been downsized from its Saturn V days to match the diameter of the shuttle’s propellant tank, which was a little less than 28 feet across.
On the day I visited Michoud, the core had reached its full length—the hydrogen and oxygen tanks had been joined by the intertank section; the topmost forward skirt, which joins the core stage to an upper stage and houses the all-important flight control computer, had been connected; and the engine section had been bolted on at the bottom. One of the four engines had been eased through a hole in the fairing, and preparations were under way for adding another engine within a week. While one worker has compared the buzz of activity to ants swarming at a picnic, when I visited, a more apt comparison would be termites, since most of the activity that day was on the inside. Work was also under way along scaffolds at the midsection, preparing the “system tunnels” that run alongside the hydrogen tank to shield oxygen feed lines and cable runs. Two massive blue rings surrounded the core, which allows Boeing and its subcontractors to rotate the entire assembly.
While the reused main engines appear almost identical to the ones that flew on the shuttle orbiters, there are important differences. NASA wants a higher power output from the engines, with a second hike planned later. That means higher pressure in the combustion chamber. “I hear comments about how NASA is using ‘old tech’ on SLS, but the [space shuttle main engine] is really different from 25 years ago,” said Steve Wofford, manager of the Marshall Center’s SLS liquid engine office. “And it was ahead of its time to begin with. It’s the best in class by a mile. SSME was upgraded five times during [the shuttle period].”
The SLS inherited 16 of the sturdy powerplants from shuttle shelves, and the first four SLS flights, consuming four engines each, will exhaust that inventory. “The next challenge for us is taking a great engine—small, lightweight, powerful, durable—how can we make that more affordable?” says Wofford. “We’re doing that right now.”
New replacement engines, NASA believes, will cost just two-thirds as much to build as the old ones. The agency is able to make use of technology that was unavailable when the SSMEs were built, such as additive manufacturing, also known as 3D printing, which can speed the manufacture of complex and demanding main-engine components. According to Wofford, in one component alone, 100 welds were eliminated.
One immense design challenge was making sure the engines and their supporting equipment could tolerate the new “base heating environment.” The SLS relies on a dense pack of hot devices, all exhausting at the same level. “We have four engines shining on each other that are co-planar with the solid rocket booster [exhausts],” says Wofford, “and there’s also an [auxiliary power unit] exhausting there.”
The shuttle had only three main engines, and their nozzles were positioned 14 feet higher than those of the solid rocket nozzles. The solid rockets throw out exhaust gases hot enough to vaporize steel, and the plume expands with altitude. Analysts decided that structural bands on the main engine nozzles and drain lines needed extra protection. “We tacked on insulation batting, and also used an ablative layer on top of the batting,” says Wofford. Batting is a space-age quilt: reflective on the outside with a thick filling of heat-resistant silica fiber.
“The [liquid] engines had to adapt and we did too,” said Bruce Tiller, manager of Marshall’s SLS solid-booster office. “We modified the thermal curtain [a ring of insulating blanket between the SRB nozzle and the aft end of the booster’s steel case] and added reflective material on the side where the engines are and added insulation there.”
The major physical difference between the shuttle and Space Launch System solid boosters is the fifth segment added to the four-segment shuttle booster. Adds Tiller: “Avionics and electronics that control the booster, the pyrotechnics that set off the separation charge, and [nozzle] steering—that’s all new.”
All these changes have been proved to NASA’s satisfaction using computer runs and individual engine firings on the test stand, but nothing yet offered by computational fluid dynamics matches the fury of the Green Run test-fire scheduled for Stennis this spring. Boeing’s Steve Ernst commented that in the end, “One good test is worth a thousand expert opinions.”
The first trial is getting the 100-ton core stage there. It will travel horizontally by transporter and barge, supported at each end, until raised by a pair of cranes and slotted into Stennis’s B-2 Test Stand. Nothing happens fast while shifting such a load. “We call it the golden egg,” said Chris Cianciola. “It’s the only one we have now; if we break it, we have no more on the shelf.”
The Green Run is a critical test, but it is not the toughest challenge the Space Launch System faces. Perhaps the biggest uncertainty in SLS development is the risk that ambitious—and highly publicized—private-sector competitors will pull ahead in the heavy-lift space race, leaving the SLS as a dry branch on the evolutionary tree. SpaceX founder Elon Musk announced development of the Falcon Heavy in 2011, the same year the SLS program got under way. Designed for reusability, the Falcon Heavy has already launched three times, the first in 2018. It can boost 64 metric tons to low Earth orbit. The first version of the SLS, on the other hand, is rated at metric 70 tons, but its first launch won’t happen until 2021, and Musk says his next rocket called Starship—a big booster topped by a shiny spacecraft with second-stage engines—will launch this year, though a prototype was damaged in a recent test, and work appears to have slowed.
The Starship, claims Musk, will carry at least 100 tons to low Earth orbit at a fraction of the cost of the advanced SLS. As former astronaut Ed Gibson told a Politico interviewer in November, “SpaceX could be a lot cheaper than what we’re going to do with the SLS and maybe even the Orion, as nice as those programs are. People have fallen in love with them, but they got old and expensive.”
According to an October 2018 audit by NASA’s Inspector General, the SLS core stage has missed its original launch deadline by almost four years. (NASA had even considered canceling the SLS Green Run to speed things up.) The report also predicted that the core stage will be as much as $2.7 billion over budget. Originally predicted to cost as little as a half-billion dollars per launch, a single SLS trip was estimated in December—by NASA administrator Jim Bridenstine—to cost between $800 million and $1.6 billion, slightly less than the IG estimate of $2 billion, not counting fixed costs. The Space Launch System did receive some support from witnesses before a November House space subcommittee hearing. One of them, former Goddard Space Flight Center director Tom Young, argued that NASA’s role in moon and Mars exploration should be leading and managing, as it did with the Saturn V and Apollo, and not merely renting rides on heavy-lift rockets created by vendors.
Meanwhile, NASA says the SLS can and will get someone to the moon by 2024. “The fact of the matter is, the only system we have today that is designed, purpose-built, to go ahead and get men to the moon and women to the moon is the SLS,” said Doug Loverro, NASA’s new associate administrator for human spaceflight. He told a staff town hall at NASA headquarters in December, “That program is absolutely mandatory....”
A lot is riding on the Green Run.
The B-2 Test Stand at the Stennis Space Center looks like an unfinished skyscraper. Its rectangular steel frame, known as “the battleship,” dates from Apollo. NASA retrofits the stand for each new rocket: At its zenith is the extension built to accommodate the SLS core stage.
Once the core stage is installed, the test will do more than assess how the four main engines perform when fired together. It will also evaluate how the new tanks and piping handle a full load of super-cold hydrogen fuel and oxygen. One of the steps in the Green Run is a “wet dress rehearsal” to verify integrity. Each tank will be filled, electronically sniffed for leaks, then emptied.
The tanks have already gone through a battery of stress tests, including checks with liquid nitrogen, but that’s toasty warm compared to the liquid hydrogen that will be pouring into the lower tank. “We have to plan for the worst case,” says B-2 test director Ryan Roberts as the elevator takes us to the top deck of the battleship, Level 18.
That plan includes a super-powered, water-deluge system fed by a battery of 11 pumps drawing from a nearby reservoir. These can deliver 5,000 gallons of water per second to the base of the stand, where it will feed tens of thousands of holes lining the flame bucket, creating a protective film of vapor. Water is also available for the giant fire-extinguishing pipes and nozzles above, aimed at the core stage and the structure alongside. “Those are only used in case of a bad day,” Roberts says. One contingency is a cryogenic liquid spill. Without the deluge system to disperse it, the contents of a breached propellant tank could weaken the stand’s structure or even worse, produce a giant cloud of explosive vapor. That’s why the nearest viewing spot considered safe for VIPs and the press during the Green Run will be a parking lot a mile from our vantage point atop the B-2.
At various facilities across the United States, all of the avionics boxes were tested for their tolerance of vibration, shock, thermal extremes, and electromagnetic effects. At Marshall, they were linked together to check for their ability to communicate and cope with a wide range of simulated emergencies. During the Green Run, according to Lisa Espy, NASA lead test engineer for core stage avionics, of special interest will be how heritage hardware—equipment adapted from earlier space ventures—holds up. One item of interest is the SLS flight computer, which has been modified from a Boeing box designed for satellite launches. Because the flight computer is crucial to a good flight, and because it’s considered more delicate than other gear, it gets to ride in a (comparatively) quiet section of the core stage, the forward skirt at the top.
Automatic controls that govern the main engines are also new. The shuttle versions relied on electronic controllers for which parts are no longer available, so the agency substituted a newer controller, one designed for the Ares rockets, and redesigned it to be compatible with the SLS main engines. The SLS has also inherited from the shuttle a health monitoring system better able to avoid the false alert that shut down an engine during climb-out on shuttle mission 51-F, which had to settle for a lower orbit than planned. Sensors and the health monitoring system were upgraded as a result.
All of these electronics as well as the pumps and engines will operate during the Green Run for the duration of an SLS ascent to orbit—just over eight minutes. (The test will be considered successful, though, if the system runs for at least five minutes.)
If the Green Run is deemed unsuccessful, the core stage could require an additional test firing. Technicians will pore over the results; check for damage from heat, noise, and vibration; and determine whether another test is necessary. “We really, really hope it works the first time like it’s supposed to,” says Roberts.
After testing is complete, refurbishment of the core stage at Stennis will take two months. Then comes a second journey by barge—to the Kennedy Space Center in Florida. At Kennedy’s Vertical Assembly Building, technicians will work for six months to integrate the stage with the rest of the rocket and Orion. Finally, Artemis I, with its cargo of 13 cubesats and an empty Orion capsule, will be launched in 2021.
During research for this article, I asked NASA engineers and contractors how they’d compare the scrupulous details of SLS core-stage testing to techniques the private sector is using. They said they didn’t know, even though SpaceX and Blue Origin are leasing some of NASA’s test stands. SpaceX investors like to describe Elon Musk as a Silicon Valley-minded disruptor willing to move fast and break things. Put NASA down as a wary organization with a hallowed track record that includes past heartache. It moves slowly and doesn’t want to break anything…unless it’s part of a test on that long, hard journey back to the moon.
NASA's New Megarocket
A little bit Shuttle, a little bit Saturn V.
The Space Launch System carries its payload—the Orion space capsule—atop its fuel tanks, as the Apollo program’s Saturn V did, but its propulsion system is inherited from the space shuttle. Engines, boosters, and tanks are all shuttle hardware, but they are supersized. There are four liquid-fuel engines instead of the shuttle’s three, five fuel segments in each of the solid rocket boosters instead of the four that made up the shuttle strap-ons, and much bigger tanks. “The hydrogen tank for SLS is almost as big as the entire External Tank on the space shuttle,” says Mike Alldredge, who leads the thermal protection system team for the SLS core stage at NASA’s Marshall Space Flight Center. Standing 177 feet tall and burning six tons of propellant every second, the solid rocket boosters will provide more than 75 percent of the launcher’s thrust. On the launch pad, they will support the entire 5.75 million-pound weight of the SLS.
The SLS will grow over time. The first rockets will be able to lift 70 metric tons to Earth orbit. Because escaping Earth’s gravity requires more power, the early versions of the SLS will carry only about 26 metric tons to the moon. As a more powerful second stage is developed and a new generation of strap-on boosters offers more thrust, the ultimate model of the SLS will lift 45 metric tons to deep space, putting it ahead of the Saturn V as the most powerful rocket in history.
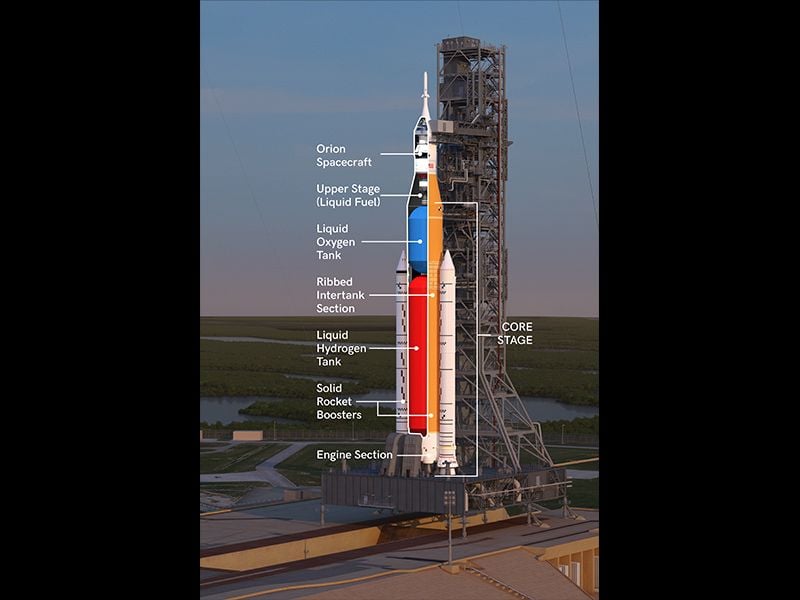