Another Way to Land on the Moon
It’s difficult and expensive to soft-land a spacecraft on the Moon, so why bother?
To our great surprise and good luck, we have found that the poles of the Moon contain significant amounts of water, most likely in the form of ground ice. What remains unclear is exactly how this water varies in concentration (both laterally and vertically), what its physical state is, and the amounts and composition of other volatiles that might be found with it. This information is crucial to the development of any plan to harvest and use this lunar water to create new spaceflight capabilities.
We have learned much using a variety of remote sensing techniques from orbit, but ground truth can only be obtained by direct measurements on the lunar surface. This requirement raises the level of difficulty. To land on the Moon following a direct trajectory from Earth, you need to slow down by about 2,380 meters per second (~2.4 km/s), a substantial amount of energy, requiring a large rocket engine and a significant amount of propellant. This rocket burn must be of precise duration so that the spacecraft gently touches down on the surface. But what if the touchdown was not-so-gentle?
We have flown impactor missions to the Moon for many years, starting with the Ranger series in the early 1960s to the LCROSS mission in 2010, whose impact kicked up a cloud of debris that was compositionally analyzed by instruments on other spacecraft. In these missions, data were collected either before the collision or by other, accompanying satellites. What if the impactor could be made to survive the collision? This possibility is something that I am exploring in terms of future exploration of the lunar poles.
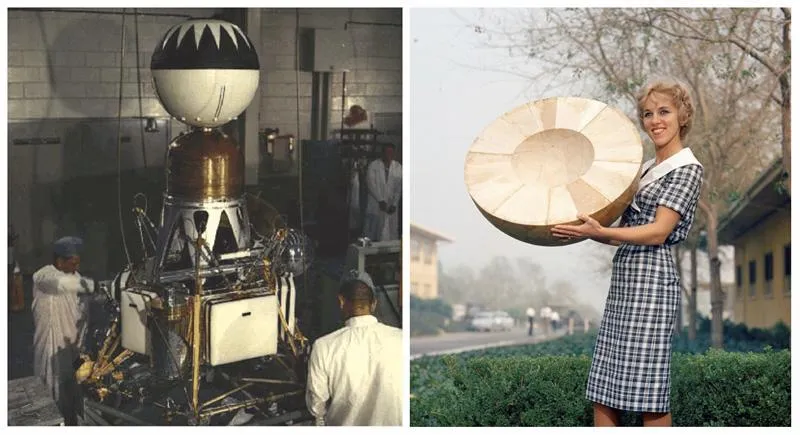
The concept of a survivable hard lander dates back to the Ranger program of the 1960s. That spacecraft was designed to take continuous television pictures as it approached the Moon, until it crashed. An early version of the Ranger spacecraft also carried a large ball designed to separate from the main spacecraft bus, fire a retro-rocket to slow down and then collide with the Moon at the relatively slow speed of about 60 meters per second, or 216 kilometers per hour (134 mph). This low-speed impact would prevent the object from being completely destroyed; encased in crushable media (balsa wood) for cushioning, the ball contained a seismometer to measure moonquakes and was designed to operate for up to 30 days. This mission design was quite advanced for its time.
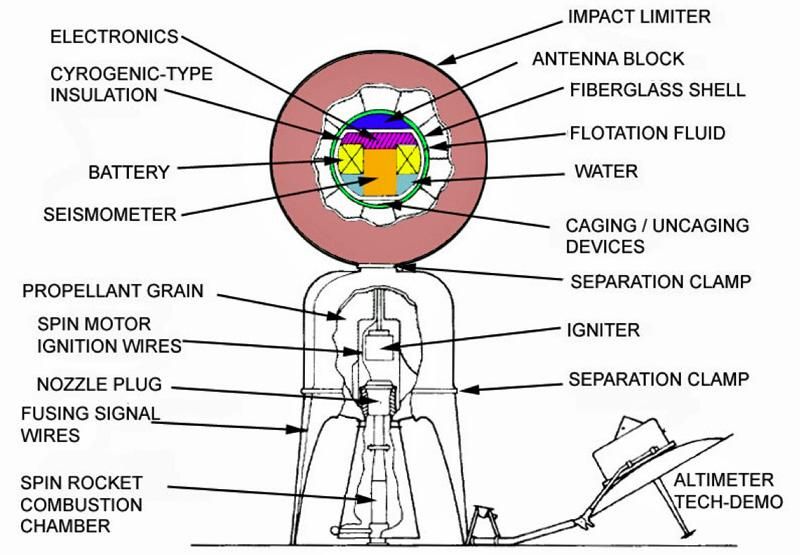
Unfortunately, the three Ranger missions that carried the ball (Rangers 3-5) all failed, although in one case (Ranger 4), the seismometer deployed and was tracked by radio as it flew over the Moon’s limb to impact on the far side. A rigorous review of the Ranger program resulted in downsizing the scientific payload, and the balsa ball crash lander was dropped from subsequent missions. Subsequent American missions (the Surveyor program) used rocket braking to gently touch down on the Moon. The success of this program created a new dogma for mission design—to make surface analyses of other planets, a soft-landing spacecraft must deliver the instrument payload.
Soft landers have much to commend them, except for their cost. A lunar soft-lander must be a complex, three-axis stabilized spacecraft, capable of independent operation in free space but with the additional requirement that it can complete a precision rocket burn to make a controlled landing—delicate and touchy, yet achievable. Once landed, such a spacecraft can only investigate a single spot on the surface (or a couple of closely spaced spots, if it can hop to another location). What if we could get close to the surface and then release a “swarm” of a dozen or more probes? We could then use a single engine burn to decelerate the probes to a manageable speed of descent and then “crash land” them all on the surface.
This is one of the mission concepts that I have been studying. I am interested in the amounts and distribution of water ice at the poles of the Moon, which is apparently widely distributed across the surface. But multiple soft landers would be very expensive (about $100 million or more per lander) and a long-lived, nuclear-powered surface rover (designed to roam widely and go into and out of the dark, polar cold traps) might cost upwards of $1 billion. However, cheap probes designed to crash land on the Moon can be made for only a couple million dollars each; for the cost of a single, powered lander, we could make dozens of these small probes. Each probe would be battery powered and would carry a neutron spectrometer, imager, radio and antenna. After landing, they would make measurements and radio the results up to an orbiting companion spacecraft before their operations stopped. This approach gives us better data coverage than a single lander—points of measurement would be distributed over a wide area.
My design mimics that of the Ranger balsa ball. However, to measure surface hydrogen, we cannot use balsa to cushion the impact (wood is mostly cellulose, a hydrocarbon that would swamp any reading of indigenous, lunar hydrogen and render the analysis worthless). Instead, we use an aluminum foam structure, which is lightweight and will not interfere with the neutron signature of lunar polar volatiles. Each sphere would have a mass of a couple of kilograms and would be designed to last for only a few hours, enough time to measure the hydrogen at its landing site and send the data up to the orbiter when it makes its next pass. The sphere would have an offset center-of-gravity so that after bouncing and rolling, it will always come to rest in one orientation, making the antenna deployment easier.
The spacecraft would carry a detachable pallet of a couple dozen probes on an orbiter. This spacecraft would be placed into an elliptical orbit with its perilune (low point) over a lunar pole. To land the probes, the pallet would separate from the orbiter just before perilune and a single, solid rocket motor would be fired to null out the orbital velocity (about 1,700 meters per second). After engine burnout, the spring-loaded probes would be released, dispersed over a wide area, and allowed to free-fall to the surface from the low perilune altitude. At a perilune of 10 km, it would take about 90 seconds for a probe to fall, hitting the surface at about 150 meters per second. Such a speed is slow enough that the aluminum foam encasing the instrument package would be adequate to cushion the shock of landing.
A probe would bounce a few times and roll downhill to some naturally stable location (e.g., a crater floor); it would then open to deploy its radio antenna. Making a surface measurement of the hydrogen concentration takes a few tens of minutes. In about two hours, the orbiter would fly over the landing sites to collect the data sent by the probes—timing and location signals and their individual hydrogen measurements—for relay to Earth. These data would provide precision ground truth to calibrate the orbital remote sensing maps and give us information on water concentrations at as many as two dozen different sites, some in sunlight and some in permanent darkness. If a few probes fail, it isn’t a critical loss—we still get a large number of distinct data points distributed over a wide area, all for the propulsive cost of only one lander (the solid rocket motor, which is discarded after burnout).
This mission design is only conceptual at this stage. The biggest advantage of such an approach is that it will give us many surface data points for the cost of a single mission. There is no need for drilling or probe burial, as neutron spectroscopy directly measures the concentrations of hydrogen in the upper meter or so of the lunar surface.
More innovative ideas for simple, inexpensive missions to get strategic data on lunar polar volatiles are needed. The more cheaply and easily we can get such data, the more likely it is that we will be able to return to the lunar surface and harvest this water for all of the useful and innovative spaceflight capability such material can produce.