A (Small) Pre-Emptive Strike Against the Doomsday Asteroid
With the DART mission, scientists try to prepare for Earth’s worst catastrophe.
/https://tf-cmsv2-smithsonianmag-media.s3.amazonaws.com/filer/18/c8/18c89fe5-277a-4a3c-991c-f3766ba9ecdb/02d_jj2021_replacesd_dart_live.jpg)
In March 1989, an asteroid twice the size of a football field careened past Earth at 46,000 miles per hour. When it crossed Earth’s orbit, it was only 425,000 miles away—about twice the distance between Earth and the moon and an uncomfortably close shave for an object that big. If the asteroid had slammed into the planet, it would have punched a hole in Earth’s crust with the force of 20,000 hydrogen bombs, excavating a crater between five miles and 10 miles wide and a mile deep. Anything within a 40-mile radius would have been obliterated, and dust flowing into Earth’s atmosphere would have cooled regional temperatures enough to affect crop growth, causing localized food shortages. If it had slammed into the ocean instead, millions of people worldwide could have been killed by the ensuing tsunamis.
NASA officials deemed the flyby a close call. And, they noted, a larger asteroid would wreak even more havoc, from civilization-rending damage to a mass extinction snuffing out entire branches of life.
The asteroid was later formally named 4581 Asclepius, for the Greek god of healing and medicine. It led to a reckoning over how to safeguard the world from harm.
Shortly after the flyby—the closest approach by a large asteroid in a half-century—Congress tasked NASA with detecting and tracking asteroids that could pose a threat. By 2010, the agency had located 90 percent of all asteroids larger than one kilometer in diameter and is still working on finding 90 percent of all rocks wider than 140 meters across.
But protecting life on Earth will require more than seeing what’s coming. It will mean eliminating the asteroid headed our way—or at least pushing it aside.
This life-preserving mission is at the heart of DART, the Double Asteroid Redirection Test, a NASA mission being launched in November. Almost a year later, when it arrives at its destination seven million miles away, the dishwasher-size DART spacecraft will fling itself into a small asteroid, which is itself orbiting a larger asteroid. The spacecraft will be consigned to oblivion, and the small asteroidal moon will shift its orbit just enough to be detectable from Earth. Scientists hope to show that punching a distant asteroid is possible, in case we ever need to move one to avert disaster.
Every space mission is full of unknowns, but this one has more than its share, from the exact size and nature of the target asteroid pair to the potential change in the smaller one’s orbit, to the size and type of the crater DART will leave behind. The spacecraft will not even see its target until an hour before it crashes into it. But what DART will beam home in its final seconds will be priceless.
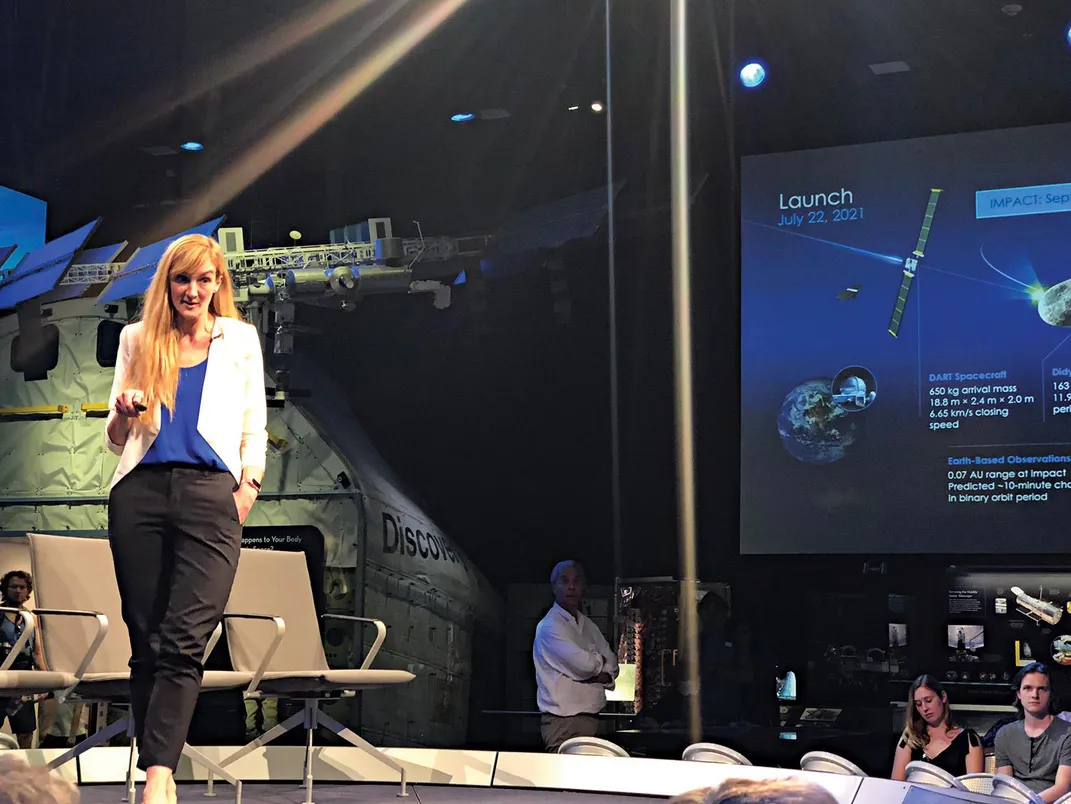
Random Rocks
Neutralizing an asteroid threat sounds simple enough in theory. With enough warning, humans could strap a nuclear warhead to a rocket and destroy a threatening asteroid well before it hits Earth. At the least, the detonation could change the rock’s course just enough to protect the planet.
“But that makes people uncomfortable for all sorts of reasons,” says Andy Rivkin, DART’s co-lead investigator at the Applied Physics Laboratory of Johns Hopkins University.
Nuclear weapons as asteroid shields were first proposed in 1969, but many scientists eventually came to favor a so-called kinetic impactor as a safer alternative and one that would not violate any international treaties. In this scenario, a spacecraft would smack into an asteroid and change its course, setting the rock on a new path that does not meet up with Earth.
But asteroids are often unpredictable, and on every mission to visit one, there have been surprises. Asteroid Eros, which the NEAR spacecraft orbited and landed on in 2001, was covered in an unexpectedly large number of boulders. Bennu, which OSIRIS-REx gently tapped in 2020, was also boulder-filled and spewing particles and gas as it traveled through the void. Asteroids are so mysterious that scientists don’t know what will happen when they nudge one. DART’s primary goal is to find out.
In 2010, the U.S. National Academies of Science, Engineering, and Medicine recommended a practice impactor mission. Andy Cheng, who now serves as DART’s co-lead investigator, realized humanity needed two asteroids in order to analyze the impactor’s effect: The impactor would strike either a partner in a binary asteroid system or a moon orbiting an asteroid. Scientists could then observe the change in the struck body’s path around the other. This realization led to DART, and the mission was funded by 2012.
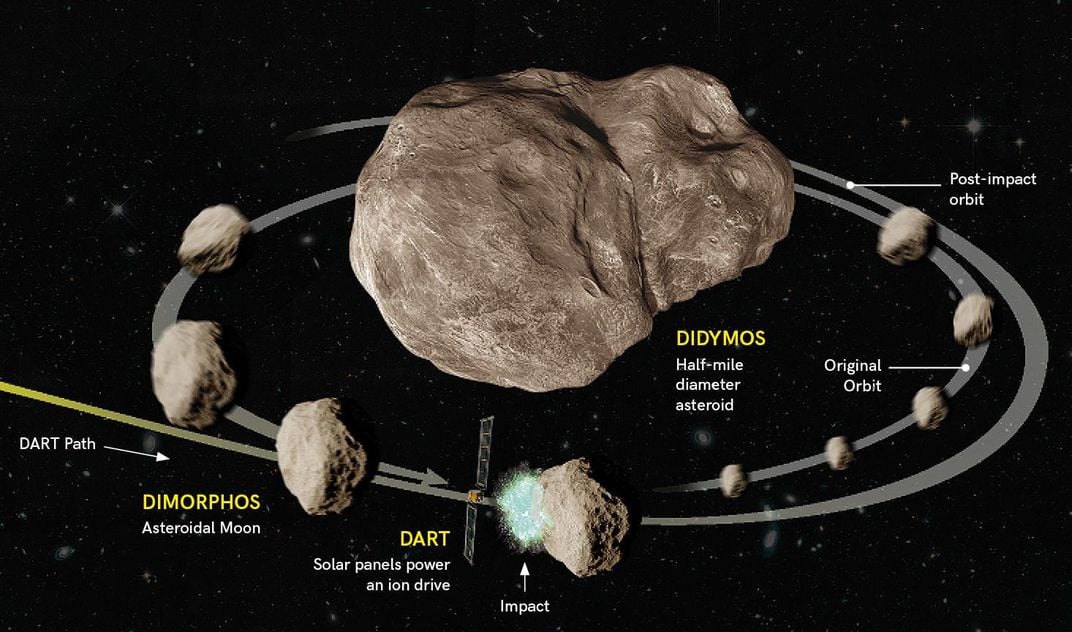
Cheng and colleagues quickly settled on an asteroid system called Didymos. The main asteroid was discovered in 1996, and its tiny moon, later named Dimorphos, was spotted in 2003. Scientists realized that the system will be closer to Earth next year than at any point in the next 50 years, which enables better Earth-based observation and tracking, Cheng says.
Hitting a moon instead of a larger main asteroid has plenty of benefits, says Rivkin. The Didymos-Dimorphos system is whizzing around the sun at 30 kilometers per second, and DART only packs a punch big enough to shift that speed by about one millimeter per second.
“In case of a real threat, that would be enough,” says Rivkin. “If you do that 10 or 20 years ahead of time, you miss the Earth.”
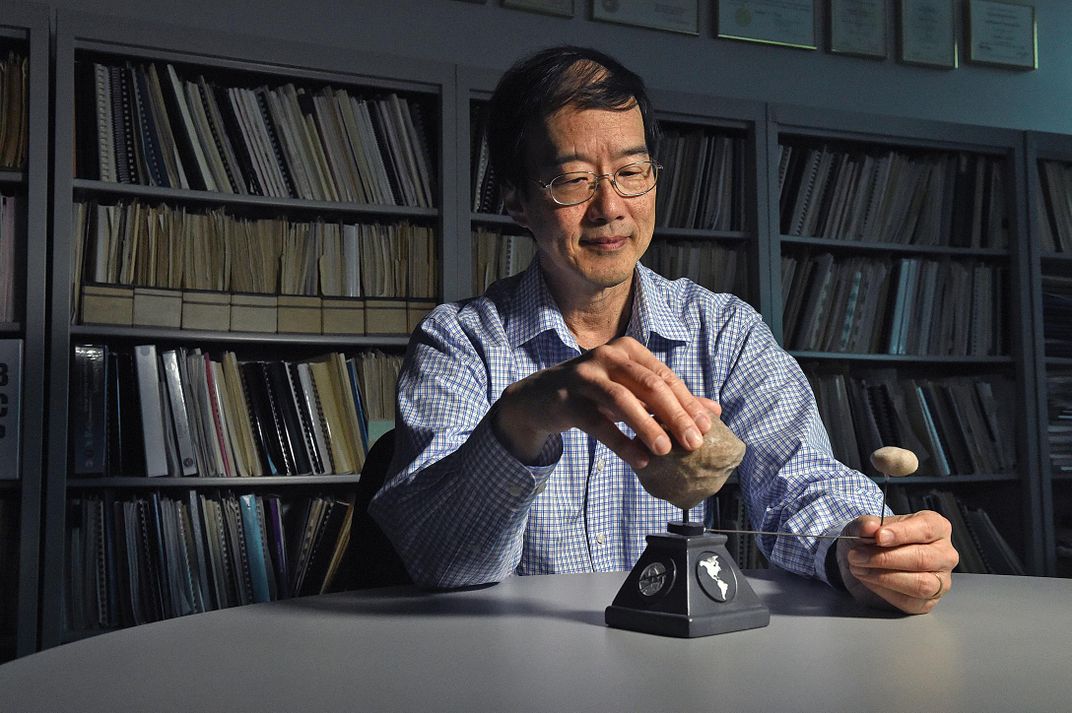
NASA’s congressionally mandated goal to find such threats means that we would probably have some warning; we already know the whereabouts of most rocks larger than a kilometer, and scientists monitor their movement using networks of automated telescopes. Asteroid location data is fed into computer software to create a digital ephemeris, which provides the position and speed of objects in space and predicts their future orbital paths.
Building an asteroid deflector the next time it’s really needed will be a little easier after the practice the DART mission provides, Cheng notes. “NASA wants to show that they can do a mission like this quickly and not too expensively,” he says.
Consider an asteroid like 99942 Apophis, a 1,100-foot-wide asteroid that could kill tens of millions of people if it hit Earth. Recent observations show it will come close but won’t hit anytime in the next century. That’s just the type of target DART is meant as practice against, Cheng says.
“In the future, if we discover ‘Oh, my goodness, we were wrong. [Apophis] is going to hit the Earth,’ we would have enough time,” he says. How long a deflector would take to build depends on just how much time lies between when scientists recognize the threat and the predicted impact. “For something as big as Apophis, which has the potential to wipe out a small country, money becomes less of an object,” reasons Cheng.
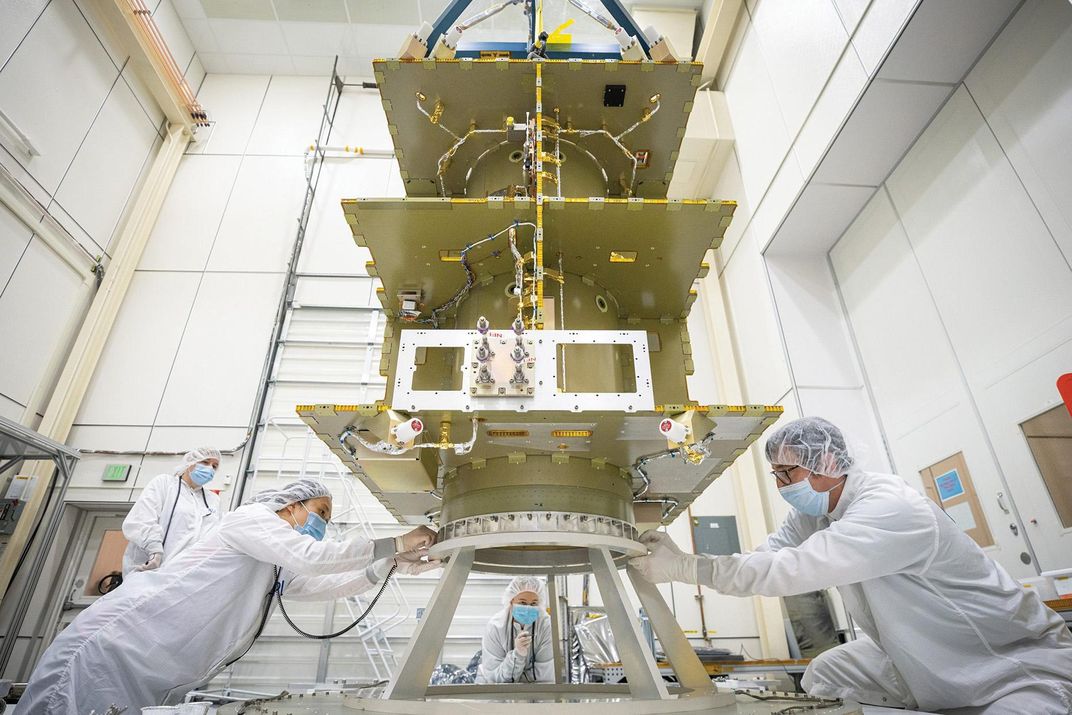
Even with sufficient time to build and launch a do-or-die mission, to nudge a real potential killer like Asclepius out of the way would require far more heft than a DART-size spacecraft.
Luckily, DART is not designed to save the day. It is designed to find out what saving the day might look like. One comforting fact about its target, though, is that it is the same composition and roughly the same size as most deadly asteroids, according to Rivkin.
“It’s representative of the kind of material that is out there and [has most commonly hit] the Earth,” Rivkin says. Dimorphos is moving around Didymos at a few dozen centimeters per second. Understanding just how Didymos and Dimorphos travel through space is one of the DART imaging team’s goals, because the only way to judge the mission’s success is to be able to measure the change in the moon’s orbit.
DART’s targets are so far away that they cannot be seen directly, so scientists on Earth will detect any orbital change by measuring Didymos’ brightness. When the moon moves in front of the asteroid relative to our location on Earth, Didymos will dim ever so slightly. If it dims earlier or later than it should per the ephemeris, the DART team will know their mission was a success.
DART will carry an Italian cubesat called LICIA, which will separate from DART before impact and capture images of its mothership’s demise. In 2024, the European Space Agency (ESA) will launch a probe called Hera to map DART’s impact crater and measure the asteroid’s mass, another thing scientists don’t yet know.
Based on observations from the former Arecibo Observatory’s radio telescope, astronomers know Dimorphos is about 500 feet wide and orbits Didymos roughly every 12 hours. They know Didymos is made of the same material as the most common meteorites. But that’s about it.
“We have no clue what Dimorphos looks like,” says Elena Adams, a systems engineer at APL. “We have some size predictions, but we don’t know if it’s a dog-bone shape, an oblong thing like Eros, or a duck-looking thing like Comet 67/P in the Rosetta mission.”
DART won’t be able to see Dimorphos clearly until about four minutes before impact. Its camera has to aim at a single pixel, barely a crumb on your phone screen. Earth-based telescopes and radar and the DART’s onboard Didymos Reconnaissance and Asteroid Camera for OpNav (DRACO) will watch Didymos and Dimorphos in the months after launch but will only be able to guess at their exact location within a range of about 15 kilometers, Cheng says. Seven days before impact, the DART team will turn on a new guidance system, built using APL guided-missile technology, and enable the spacecraft to aim itself at Dimorphos. It will have to crash within 15 meters of its aim point. Letting the spacecraft guide itself is necessary, Cheng says. NASA’s commands—sent after spacecraft imagery had been received on Earth—would not arrive fast enough to tell the spacecraft where to hit.
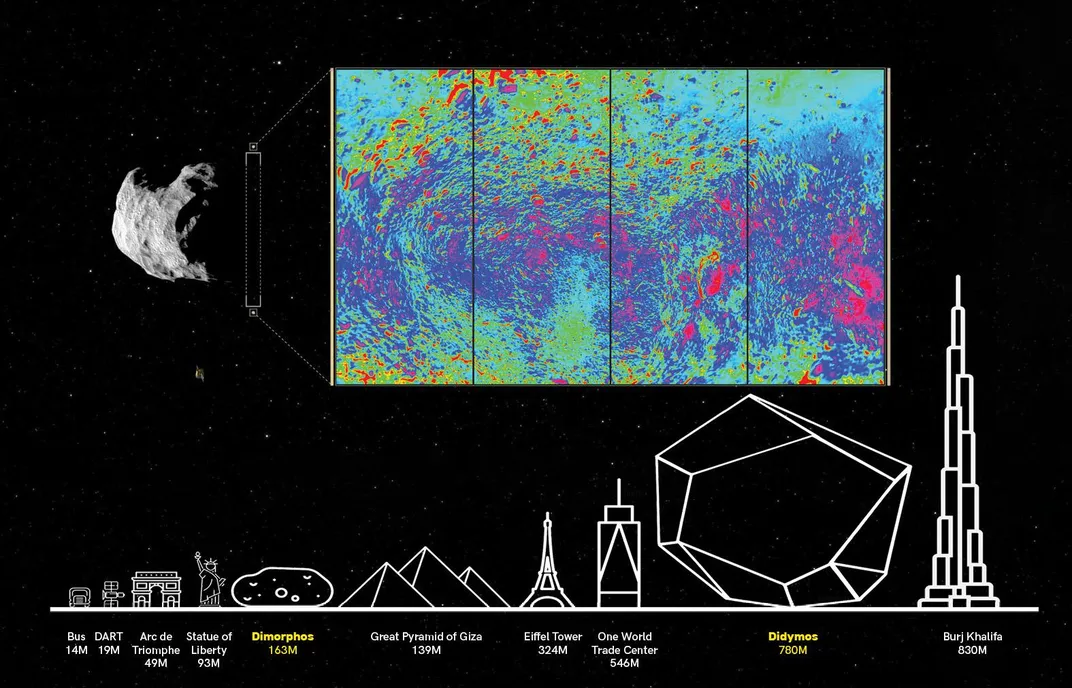
Built Like a Tank
DART will also serve as a test bed for the next generation of space equipment. Space mission planners usually care about weight more than almost anything else—every gram sent aloft must be carefully weighed against the spacecraft’s fuel requirements, design parameters, and science goals. But because DART’s 670 kilograms don’t come close to maxing out the capability of the SpaceX Falcon 9 rocket that will loft it—it won’t take much mass to nudge the asteroid—DART’s engineers were able to throw on practically anything they wanted. Other asteroid-visiting missions have complex, heavy cameras and even asteroid sample-return equipment, but not DART.
“Mass is the most precious commodity you can ever have in space travel,” says Adams. “But on DART, we’re like, ‘Eh, we don’t worry about it.’ It is built like a tank.”
What’s more, DART doesn’t have to fly as fast as the Parker Solar Probe or other deep-space missions, which means the energy required to leave Earth is a little lower. The navigation system—SMARTNav, or Small-body Maneuvering Autonomous Real-Time Navigation—is critical for DART, which will not have any images of its target until moments before impact. With no advance images, all decisions need to be made on board, with no human at the joystick, explains Michelle Chen, who leads the SmartNav team at APL.
A typical new TV has 4K resolution, meaning its screen measures roughly 4,000 pixels horizontally. The DRACO camera has only 2K, and within that, the Didymos system—both asteroid and moon—occupies a single pixel, until the mission’s final hour.
“When we are looking at the asteroid, I always have to clean my monitor screen, because I’m never sure if it’s a dust speck or what,” Chen says.
The camera can’t resolve both objects separately until about an hour before impact. So SmartNav’s algorithm continually scrutinizes DRACO’s images, filtering out other objects and dust, to lock onto its target.
Chen has spent the past several years testing her algorithm to make sure it can handle any surprises. If DART arrives at Didymos and finds it has more than one moon, SmartNav will know what to do. It can handle unexpected lighting conditions—if the moon is opposite the sunlit side of the asteroid, for example, and therefore harder to spot. Even though SmartNav will not survive DART’s destruction, Chen says its breakthroughs will inform new systems for the next generation of spacecraft. This is possible only because DART has an ordinary central processing unit-powered computer as well as a field programmable gate array, or FPGA, which can handle specific tasks with great efficiency. The FPGA will allow DART to handle several tasks simultaneously, including streaming images to Earth in its final moments, processing those images for SmartNav to use to pilot the craft, and firing its hydrazine thrusters to adjust its trajectory.
The burden of ultimate success or failure rests on the shoulders of the mission design team, who must figure out the spacecraft’s approach geometry, make sure its antennas are pointed the right way to communicate with Earth, ensure that its prototype solar panels don’t wiggle the craft too much, and check that DRACO is pointing the right way to lock on to its target.
DART has two propulsion systems to make sure it is in the right place at the right time. Only one will be used for critical guidance and is a traditional spacecraft thruster system, with 12 small engines using the common rocket propellant hydrazine. But DART will also carry a new electric ion propulsion system called NEXT-C. This ion drive uses electricity harvested from DART’s enormous solar panels. They are unique among spacecraft but may be a game-changer for future probes because they’re so lightweight. The arrays are built of a flexible material, which unfurls after launch and stretches between two rigid booms on each side of DART. “You have this rolled-up thing that looks like a sausage, and then after launch, you actuate the mechanism and snap it open, like a snap bracelet,” Adams says.
The ion drive powered by the array works by knocking electrons free from a gas propellant to make ions. The positively charged gas is repelled by a negatively charged electric field. The ions are discharged from the engine, pushing the craft just as a typical exhaust would.
Though the ion drive won’t produce much thrust, it’s more than DART will need, says Justin Atchison, an engineer at APL. The real benefit is its ability to shift gears, as it were, through a wide range of power levels. It can use a range from 600 watts up to 7.5 kilowatts. It has a much wider throttle zone than other ion drives and is much more efficient than typical thruster systems.
“You can use the same thruster when you are near the sun and have high power or far from the sun and have low power,” Atchison says. This will enable future spacecraft to adjust their trajectories and velocities no matter where they are—even though the latter is not really an issue for DART.
When DART arrives, it will have very little time to assess its surroundings before it shatters to bits. Many months later, scientists on the ground will still be busy reconstructing its final moments, says Angela Stickle, a planetary scientist at APL whose specialty is hypervelocity impacts. Her simulations will help scientists understand what Dimorphos is like and maybe learn more about how binary asteroids form.
Although binary asteroids are common—they represent one in every six asteroids—scientists are still unsure about how they came to be. The asteroid’s moon might have calved off from Didymos at some point in the past, either through centrifugal forces or an impact with a different object. Or it’s possible that Didymos captured a small asteroid crumb that was itself calved from a larger object.
Stickle says she is eager to learn more about the moonlet, which will be possible by studying its change in trajectory after impact.
Although ESA’s Hera probe won’t arrive for a few years, the nature of DART’s demise will tell Stickle and her fellow scientists plenty about the rock that destroyed it. DART’s own imagery will show what Dimorphos looks like in the few seconds before impact, and the Italian cubesat, LICIA, will watch the ejecta. Then astronomers like Cristina Thomas, who works at Northern Arizona University and leads DART’s observation working group, will scrutinize the change in Dimorphos’ orbit. Thomas has applied for time on the James Webb Space Telescope to check Dimorphos’ new trajectory, and she is developing software that can pull tiny glimmers of light from telescope observations to see how the light changes. “We draw a circle around it, pull out all the light in that circle, and we can see these small changes,” she says. “If it was just you and me looking at it, you wouldn’t be able to see anything, but the computer can see.”
Stickle can plug all this data into her calculations and come up with new results on the nature of the asteroid. But the asteroid still might throw a wrench into everyone’s plans.
“I more worry that it’s going to be some crazy new asteroid structure that we’ve never seen before,” she says. “We did some experiments where we shot into cotton candy, and the whole thing just explodes. I don’t think that’s likely for an asteroid, but it could have a weird structural material property that we just didn’t predict. People are creative, but asteroids have proved us wrong in the past. And space is weird.”
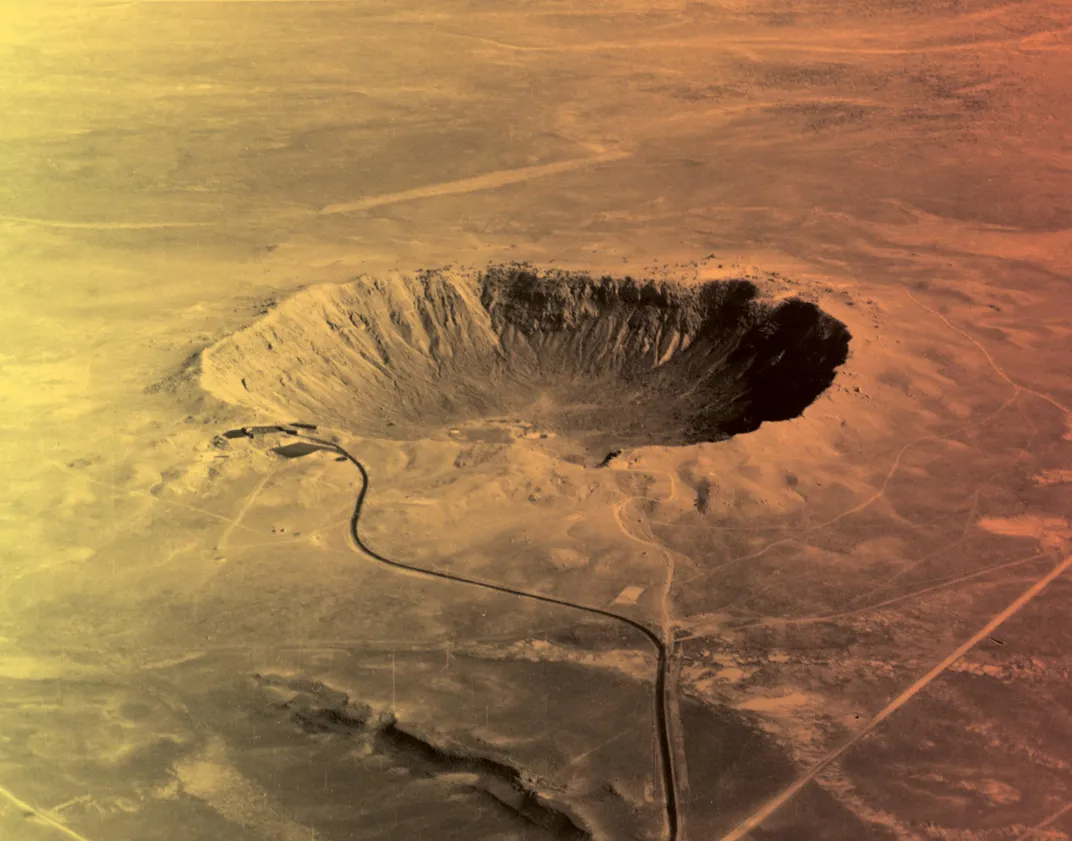
Deadly Precedent
“I thought, ‘I’m going to spend all these years doing something that’s literally going to puff up in smoke,’ ” Chen recalls. “But to me, it’s really a stepping stone.” Atchison says he likes the project’s finality. The mission has one, specific task, and the team will know without a doubt whether they pulled it off.
“That part is what keeps me up at night,” Atchison says. “Making sure we get it right the first time, because we don’t get a second time.”
That may be true for Earth too. We know a deadly strike from space has happened before. In 1989, geologists confirmed a massive crater off the coast of Mexico’s Yucatan Peninsula. Throughout rocks on Earth, scientists found layers awash in iridium, an element known to come from asteroids. The iridium spike matched the Cretaceous-Paleogene boundary—the demarcation of the downfall of the dinosaurs, in an extinction event that wiped out almost all life on Earth.
What came to be named the Chicxulub crater proved that space rocks can end the world. Now it’s up to DART to prove that spacecraft could, one day, save it.