Big Glass and the Age of New Astronomy
The fight to put a monster telescope on Mauna Kea is part of a bigger war looming among astronomers.
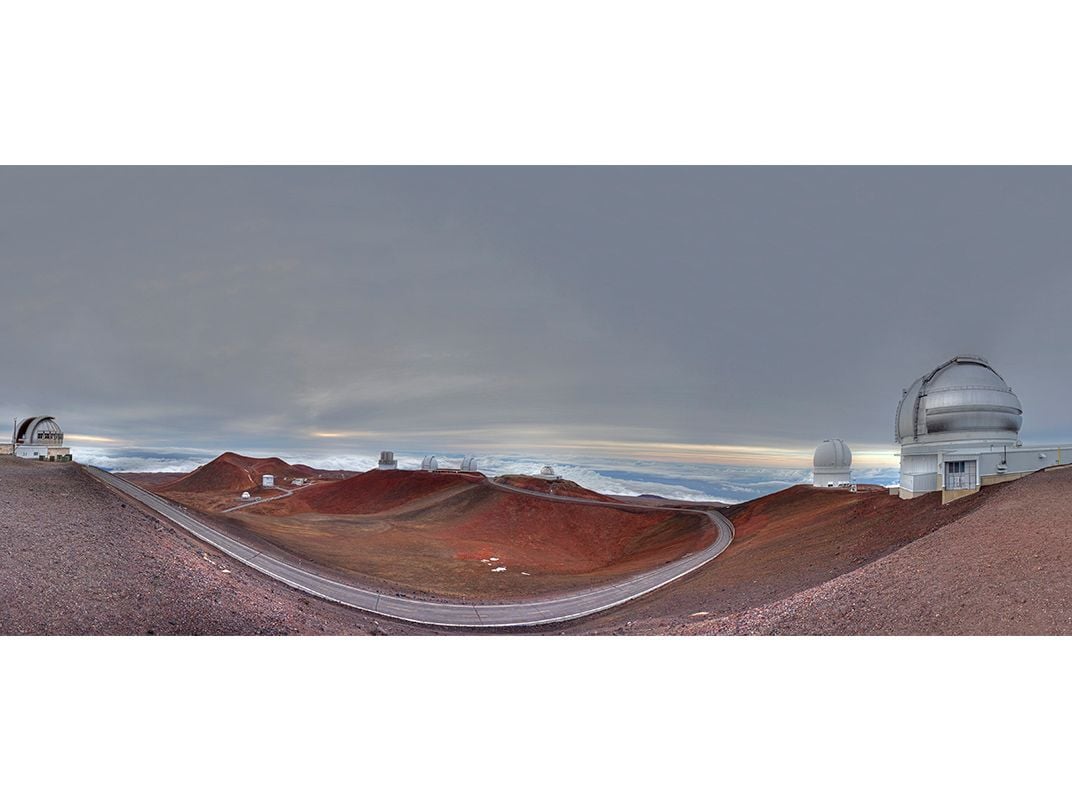
The tallest island mountain in the world is Hawaii’s Mauna Kea, where the thin atmosphere and absence of light pollution create some of the best observing conditions for astronomers. At the summit, 13 telescopes sit along a ridge of formations that have built up around volcanic vents. The oldest telescope on site, and still the smallest, is the University of Hawaii’s 2.2-meter (7.2-foot) UH88, built in 1968. Mauna Kea is best known as the home of the twin 10-meter Keck telescopes, which saw first light in the 1990s and remain two of the largest optical and infrared telescopes in the world. Collectively, this baker’s dozen of observatories has dominated ground-based astronomy for four decades. But recently, Mauna Kea has become embroiled in a dispute that could radically alter the future of astronomy, and serve as a cautionary example of what we might lose if it keeps going down this path.
In 2009, Mauna Kea was chosen as the site for the Thirty Meter Telescope, a mega-observatory proposed by the California Institute of Technology, the University of California, and national science agencies in Japan, Canada, India, and China. Its massive mirror will be made from 492 segments and have 81 times the sensitivity of the Keck telescopes. Ed Stone, a Caltech physics professor and the executive director of TMT (not to mention former director of NASA’s Jet Propulsion Laboratory), explains why scientists are pursuing a telescope more than three times the size of the biggest one currently on Mauna Kea: “If you want to see the very first stars in the universe,” he says, “you need a telescope of this class.” Keck has been able to observe a galaxy that existed about 570 million years after the Big Bang, but it just isn’t capable of observing the most distant stars, the first ones, which formed about 400 million years after the creation of the universe.
“Another frontier that needs the collecting power of a new generation of instruments is the study of exoplanets,” Stone says. “Our challenge is developing the technology and capability to study those planets—for instance, to determine whether microbial life might have evolved on them.” These are the types of fundamental questions the TMT should be able to address. And yet, the giant telescope may never be built.
In October 2014, as officials and construction crews headed to the site for the ground-breaking ceremony, a group of native Hawaiian protesters blocked their access to the summit and refused to move until the project was stopped. Native Hawaiians consider Mauna Kea a sacred site—many generations have returned to the mountain to bury the piko, or umbilical cords, of their newborn children (piko also means “mountain summit” in Hawaiian)—and have been vocal in their opposition to building observatories there for years. Hawaii’s governor, fearing violence might break out, negotiated a temporary halt in the construction. Then, last December, the state’s supreme court vacated the observatory’s building permit, sending the application back to the land and resource agency for a new hearing. Though the telescope’s managers still hope to build on Mauna Kea, they also fear a long legal battle that they will eventually lose, and have started to seriously consider sites in Baja California, the Canary Islands, Chile, India, and China.
Some Hawaiians are also fighting the extension of the entire observatory complex’s 65-year lease on the summit, which expires on December 31, 2033. Doug Simons is the director of the 3.6-meter Canada France Hawaii Telescope and former director of the Gemini Observatory, which has twin eight-meter telescopes on Mauna Kea and in Chile. He says that without assurances that the master lease will be extended, the agencies that fund the observatories will be reluctant to invest in improvements or new instrumentation. In fact, some observatories had already begun to change operations in preparation for the Thirty Meter. TMT’s Japanese partner operates Mauna Kea’s eight-meter Subaru telescope, and had started winnowing its instrumentation so that it operates exclusively as a wide-field telescope in collaboration with the new arrival. “They haven’t gone so far down the path that it’s irrecoverable,” Simons says. “But they have taken the most steps of all the Mauna Kea observatories in advance of TMT’s arrival.” If the giant telescope isn’t built, Subaru will have to reconfigure again to remain a meaningful contributor to astronomy. And if the native Hawaiians succeed, the entire scientific complex will be dismantled, and the land returned to the state.
The controversy surrounding the TMT and its impact on the other Mauna Kea observatories seem like a local story, but the struggle is also symbolic of a broader problem. Over the past few decades, the field of astronomy has been dominated by efforts to build newer and bigger telescopes. The TMT is projected to cost $1.4 billion, and the other observatories being built in this wave (all three in Chile) come with similar price tags. The Giant Magellan Telescope, which will actually house seven 8.4-meter telescopes, will likely exceed $1 billion. The staggeringly huge 39.3-meter European Extremely Large Telescope, or E-ELT, is projected to cost $1.35 billion. And the relatively dainty 8.4-meter Large Synoptic Survey Telescope (LSST) will cost $650 million to construct, but the bill goes up over $1 billion when it includes operation for the 10-year sky survey the instrument is projected to start in 2020. “A billion dollars is pretty much the entry fee into this particular game,” says Shrinivas Kulkarni, Caltech’s director of optical observatories.
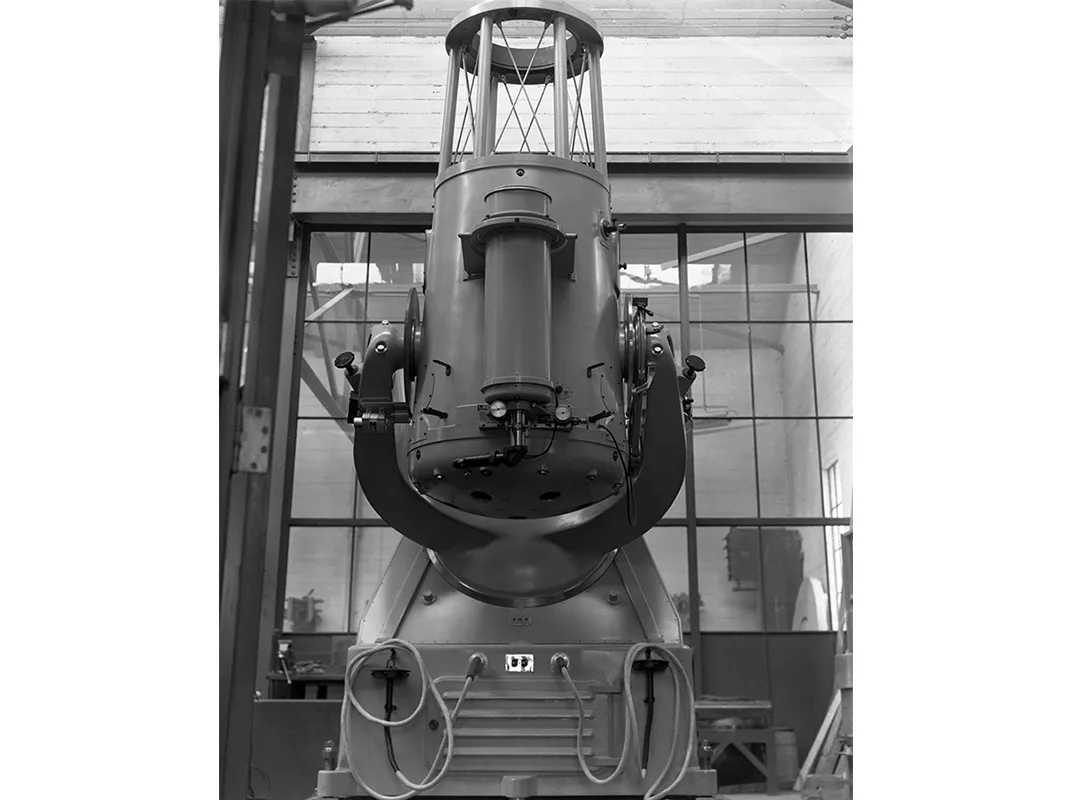
Because Caltech is one of the primary partners of the Thirty Meter project, part of Kulkarni’s job is to oversee that investment. But even though he strongly supports building the observatory, he believes the trend toward these massive and costly projects represents a sea change in how astronomy is practiced. Increasingly, Kulkarni says, the study of the universe has become the province of physicists—especially particle physicists—and they look at the world very differently than astronomers do.
“Astronomers used to be the phenomenologists of the universe,” Kulkarni says. “Just as a plant biologist studies plants of various sorts and a zoologist studies various sorts of animals, an astronomer does the same thing for the universe. We go and look for stars, for galaxies, for intergalactic media, and we catalog them. We see how the energy formed; what’s the life-cycle of stars; what’s the end product; what’s the ecosystem. You could almost regard astronomers, like zoologists and biologists, primarily as explorers, as catalogers and explainers. That’s what we do.”
But two things changed that, he says. The first was the discovery of cosmic background radiation in the 1960s. This radiation, essentially the first light in the universe, dates back to just a few hundred thousand years after the Big Bang. In 1989, NASA launched COBE, the Cosmic Background Explorer, which enabled astronomers to begin seriously probing its nature. COBE and several subsequent spacecraft, including Europe’s ongoing Planck mission, have mapped the distribution of this radiation across the universe. “The discovery of background radiation showed the real link between astronomy and basic physics,” Kulkarni says. Even though the discovery was largely driven by the techniques of astronomy, it fell to physicists to explain the high-energy environment right after the Big Bang. Suddenly the entire universe was a laboratory for particle physicists.
This blending of physics and astronomy was initially a boon to both fields. Kulkarni points out that it was a theoretical physicist, Alan Guth, who came up with the idea of cosmic inflation, one of the central ideas of modern astronomy.
The second big change in astronomy was the discovery of dark energy. In 1998, two groups of astrophysicists studying supernovas found evidence that the universe wasn’t just expanding, but expanding at an accelerating rate. This Nobel Prize-winning discovery led to the postulation of a form of energy that would explain the outward force. Dark energy is now believed to account for more than 68 percent of the energy and matter in the universe. (Dark matter makes up 27 percent. Ordinary matter, the stuff you can see and detect, makes up the rest: less than five percent of the universe.)
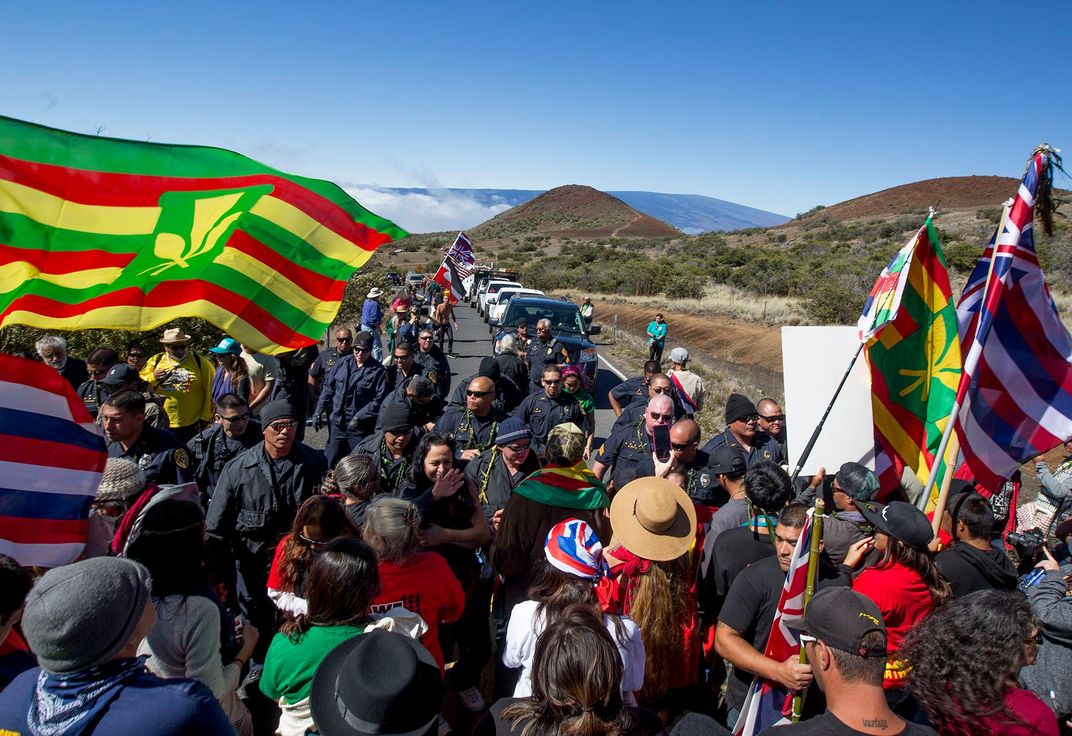
These discoveries advanced our knowledge of the universe tremendously. But they also forced physicists and astronomers to learn how to live together, says Kulkarni. “The culture of particle physics is different,” he says. “As much as astronomers are phenomenologists—explorers who want to see the breadth and diversity in the universe—physicists are the exact opposite. They’re what we call reductionists. They try to reduce the complexity of the observations of phenomena into as few principles as possible.” The whole body of what we now call mechanics derives from Newton’s second law of motion: Force equals mass times acceleration. The predictive power of this kind of theorem has made physics the king of sciences, Kulkarni says, and mathematics the queen. Unlike astronomy, the basic impetus of physics isn’t to discover something new; it’s to develop theories to explain known phenomena, then create experiments to test those theories. Because of the growing influence of physics, the discipline’s method is steering the entire field of astronomy in that direction too.
Today, an enormous amount of money is being spent on these grand experiments—many of which are similar. “The Europeans are launching the space mission called Euclid, which, as the name implies, is looking at the geometry of space,” says Kulkarni. “Not to be outdone, in 2025, the U.S. is launching something called WFIRST, which will also produce a geometry of the universe. And one of the main goals of LSST is to measure the geometry of the universe. So there’s been an enormous investment of money into these very large, high-profile, almost singularly focused, fundamental experiments.”
Those observations are bound to teach us a lot, but as Kulkarni sees it, the facilities required to do the experiments are of such a scale that they limit the amount of other types of astronomy that can be done. Lost in all this will be the serendipity that comes from basic exploration.
Although other astronomers may disagree with Kulkarni about the severity of the problem, his basic premise isn’t particularly controversial. Especially coming from someone at Caltech, says Doug Simons: “Look at the history of Palomar [Observatory] and Caltech, which has dominated the field of astronomy like no other school. Who discovered quasars, for example? That was substantially done at Caltech and Palomar. Nobody even knew that such objects existed in the universe…. We know now that black holes power quasars, but when [Dutch astronomer Maartin] Schmidt identified the first quasar, QC273, it was a completely unpredicted product of innovative observing at Caltech. That’s what [Kulkarni] is talking about: finding things you never knew or even imagined existed in the universe.”
But not everyone agrees with the notion that big physics-based projects remove the chance for the serendipity that Kulkarni yearns for. Ed Stone refutes that idea by pointing to the first detection of gravitational waves at the Laser Interferometer Gravitational-Wave Observatory, or LIGO. “I think that many astronomers now believe—once one was detected for the first time, and the merging black holes that caused it were determined to be much more massive than the models suggested. That means we’ve learned something else about nature besides just confirming Einstein’s theory of gravity. So in many cases, an experiment starts out answering a physics question, but then begins to answer questions having to do with astronomy and astrophysics.”
Even if you make the case that massive, narrowly focused observatories like LIGO and TMT contribute to basic exploration, building them still affects the rest of the field. The budgetary burden will fall on the existing stable of smaller, older telescopes. Certainly that’s the case on Mauna Kea, where part of the bargain to build Thirty Meter included shutting down some of the older facilities. To free up operational funds for new, larger facilities, the 2010 Astronomy and Astrophysics Decadal Survey recommended closing 84 older ones.
This, of course, seems like a perfectly sensible attempt to prioritize projects in the face of limited resources. “Sometimes there is no other way than to build a large instrument,” says Stone. “You just have to very carefully choose which ones you decide to do next. That’s why we have the Decadal Surveys, which the National Academy of Sciences does to advise [the National Science Foundation] and NASA.”
The decision to shut down smaller, older facilities may make sense for budgets, but not necessarily for science. Rene Walterbos, who chairs the board that oversees the Sloan Digital Sky Survey, points out that unlike the big facilities that do particle physics research, telescopes don’t become obsolete as they age. After the Large Hadron Collider was built at CERN in Geneva, Switzerland, Fermi lab’s Tevatron particle accelerator in Illinois was shut down because no new research could be done at its lower energy levels. That’s simply not the case for telescopes, Walterbos says. “In astronomy, any telescope can keep observing. People still find useful things to do, even with very small telescopes.”
If you’ve ever used a camera, you know that the more you zoom, the narrower your field of vision becomes. The small telescopes of the world may not see as far as their modern 10-meter siblings, but they see wider. That makes the older generation of telescopes better suited for broad surveys of the sky. Walterbos points out that the Sloan survey, which for the past 15 years has operated two 2.5-meter telescopes in New Mexico, has been one of the most productive astronomy programs in the world. The Sloan survey has been systematically creating three-dimensional maps of a large portion of the universe, data that’s made public and used for research at other facilities. Indeed, one of the main functions of smaller telescopes is to find new things for the TMTs and Kecks of the world to take a closer look at. At Mauna Kea this collaboration is practically a matter of walking a new finding across the street. “That’s exactly right,” says Gary Davis, former director of the 3.8-meter United Kingdom Infrared Telescope. “A lot of the discoveries at UKIRT were followed up on Gemini’s larger telescope.”
Another cost of shutting down older observatories is more straightforward: There will be fewer facilities where astronomers can do research. Many astronomy students and faculty are at universities that have no observatory; to conduct their research, they compete for time elsewhere. But many of these new, large facilities tend to be owned by groups of private universities, which give first dibs to their own scientists.
“People may look at TMT and say, ‘Well, that’s great, but we’ll never get in there,’ ” says Walterbos. “That’s a potential source for real tension. Early in the next decade, the NSF will have to figure out if they can afford to make an investment and be a partner in that and, in return, provide access to the community. Or will they not be a player at all? The pressure on their budget is severe, and they have a very difficult job—as does the community as a whole—figuring out what the balance will be.”
Another benefit of smaller telescopes is the flexibility for astronomers to be creative, says Davis, who left Mauna Kea to work on the ambitious Square Kilometre Array radio telescope being designed for locations in Australia and South Africa. “That’s where the real innovation happens, because it’s cheaper to do that innovation on small telescopes. If I want to try some weird observing mode, it’s easier to do that on a telescope that costs $1.2 million a year to operate than one that costs tens of millions.”
Nowhere is the impact of the innovation at the smaller telescopes more obvious than on Mauna Kea. Whenever the issue of old versus new comes up, Doug Simons likes to pull out a list of observatories worldwide that had the biggest scientific impact in 2015. It’s a metric one of his colleagues calculated by taking the number of publications that come out of a facility—a measure of productivity—and multiplying that by the number of citations those publications receive—a measure of influence. The twin Keck telescopes top the list, but the next two are its sub-four-meter Mauna Kea neighbors: CFHT and UKIRT, both of which have been operating for nearly 40 years.
That success, Simons says, is largely the result of a willingness to take risks, particularly on instrumentation. In 1996, CFHT was the first telescope to regularly support observations with adaptive optics, the use of a high-speed, deformable mirror to cancel out the effects of atmospheric turbulence in real time to get sharper images. “My first job after graduating from the University of Hawaii was as a resident astronomer at CFHT,” says Simons, “and I remember the ferocity of the debates back then at this ‘black magic’ technology…. It wasn’t an easy decision, but we decided to go ahead and pursue it at CFHT, and we were the first out of the starting blocks. It was a spectacular success.” No major telescope today is built without adaptive optics.
More recently, CFHT invested in the SITELLE, an instrument successfully tested last year that analyzes the spectra of every object in its view simultaneously. “SITELLE can measure the spectra of complex fields in a couple of hours, which would take conventional slit spectrometers many nights to complete,” says Simons. This is one of a handful of novel instruments at CFHT, keeping it on the cutting edge.
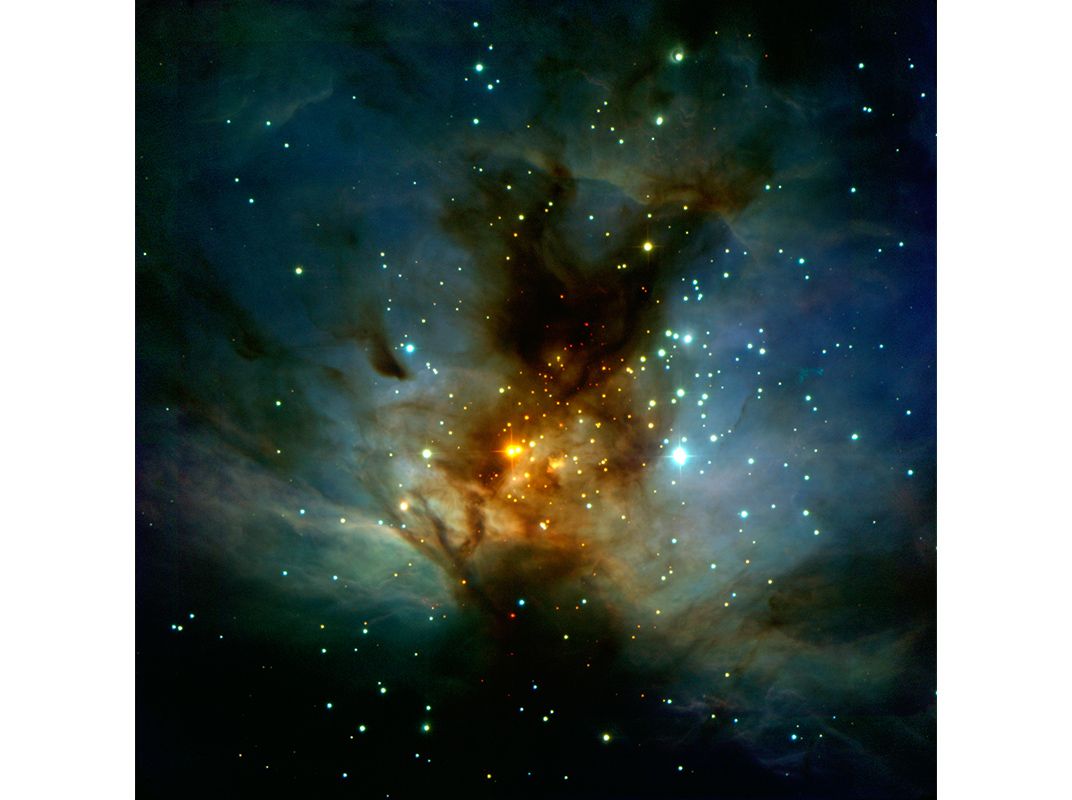
Another key innovation at the older, smaller telescopes has been automation. At Caltech’s Palomar Observatory, Kulkarni built an enormously productive research program by automating two instruments, the 1.2-meter and 1.5-meter telescopes. The wide-field survey, called the Palomar Transient Factory, has cataloged millions of supernovas and other short-lived features of the night sky. That was only possible, Kulkarni says, because of automation, which speeds up the process and frees astronomers from the mundane tasks of their research. He’s reopening the survey again next year as the Zwicky Transient Factory, using the same telescopes equipped with updated cameras.
In fact, as older telescopes endure deeper resource cuts, the only thing that will save some of them, says Davis, is embracing automation. In 2010, he automated UKIRT so that the whole operation could be run from the headquarters in Hilo, at the base of Mauna Kea. “After making the changes, our operating budget went down to $1.2 million a year,” says Davis. “On larger-aperture telescopes, it’s typically tens of millions.”
Yet, all these advantages may not be enough to save the nation’s older, smaller telescopes. Says Kulkarni, “Either you’re doing astro-particle physics—some big experiment that costs a lot of money—or you’re going to build a big telescope, like TMT, that also costs lots of money. This completely squeezes the people who use moderately sized telescopes, people that I call traditional astronomers.”
So while the Thirty Meter Telescope’s location awaits judgment—even if it isn’t Mauna Kea, the observatory will be built somewhere—a generation of explorers ponder more existential questions about their field. If the bright lights shining on Big Glass cast too much shadow on the stable of workhorse observatories, will the discoveries of astronomy fade into the darkness with them?