Today’s Hypersonic Weapons Could Lead to Tomorrow’s Mach 5 Airliners
Into the future at 6,000 MPH
:focal(739x398:740x399)/https://tf-cmsv2-smithsonianmag-media.s3.amazonaws.com/filer/98/35/9835edd5-f832-41b9-b0cc-ef3a07842f79/05m_dj2020_whiteoaktunnelvehicle_live.jpg)
Tucked away on a wooded suburban lot in White Oak, Maryland, a large, slightly rusted metal sphere rises behind one of a cluster of low brick buildings that at first glance could be abandoned elementary schools from the 1950s.
“That’s a replica of the German Peenemünde wind tunnel facility,” Dan Marren tells me, gesturing at the structure closest to the road as we meet just past the security gate. During the 1940s, German scientists led the world in developing supersonic flight, using a 40-centimeter by 40-centimeter wind tunnel in Peenemünde to develop the V-2 rocket, he says. The original German wind tunnel, now called Tunnel 1, was installed at White Oak, to be used by a dozen German scientists, who helped develop U.S. missile systems.
We’ve come a long way since then. Marren is the director of the White Oak annex of the U.S. Air Force Arnold Engineering Development Complex, home to Tunnel 9, a hypersonic wind tunnel. Whereas Tunnel 1 could produce supersonic airflows in the Mach 2 to Mach 3 range, Tunnel 9 is capable of producing airflows as fast as Mach 18. It’s the heart of high-speed aerodynamics testing in American aerospace. Whether you’re the Air Force developing anti-missile defenses, NASA working on the new Orion space capsule, or Sierra Nevada Corporation refining the Dream Chaser spaceplane, if you want your design to fly really fast, you’re probably at some point coming through the door at Tunnel 9, the epicenter of current hypersonic research.
Hypersonic vehicles have flown through the atmosphere since 1960, when the first Corona spy satellite returned its reentry vehicle. Apollo, Vostok, and Soyuz capsules, the space shuttle, and test ICBM warheads have all transited the upper atmosphere at about Mach 20. But sustained, fully controlled flight through the atmosphere at hypersonic speeds, powered by air-breathing engines, has not been attained, and Tunnel 9 has seen a lot of business in recent years as interest in hypersonics heats up both in the United States and abroad. The U.S. Department of Defense is studying hypersonic weapons systems. (Russia and China are also developing hypersonic cruise missiles.) Boeing is giving serious thought to a Mach 5 airliner. “Air-breathing hypersonics open up a region of flight that we have not fully exploited,” Hallion says: the so-called transatmosphere. He’s referring to a region of the atmosphere between about 120,000 feet and 500,000 feet—“the border area,” he says, “between flight within the atmosphere and flight into space.”
With 199 flights in the 1960s, the experimental X-15 rocketplane was the first U.S. aircraft to explore the transatmosphere. It achieved altitudes greater than 354,000 feet and a top speed of Mach 6.7. It was an enormously successful research program with profoundly limited range. For a practical aircraft with reasonable range, rockets will not work.
“If you tried to build a modern airliner and instead of using jet engines made it rocket-powered, you wouldn’t get very far,” says Mark Lewis, an aerospace engineer at the Institute for Defense Analyses and former chair of the Department of Aerospace Engineering at the University of Maryland. “The range would be severely limited because you’d have to carry all the oxidizer on board.”
But jet engines of the type found in airliners and even the highest-speed fighter jets have their own limitations, according to Hallion. A jet engine is a rotating turbine that sucks air into an inlet, compresses it, mixes it with fuel, ignites the fuel and passes the heated products out the back through a nozzle to create thrust. Adding afterburners, “you can get that to work up to about three, three and a half times the speed of sound,” says Hallion. “Beyond that point you’ve got a bit of a problem, and the problem is that the rotational machinery isn’t doing really anything for you.” (Turbojets can ingest only subsonic air. The SR-71, which reached Mach 3.2, required a ramjet to slow air down before reaching compressors.)
Solution: Get rid of the machinery. Let the high-speed air entering an engine compress itself in an engine known as a ramjet. “Basically, it’s just a tube that you inject fuel into, you ignite it, and then you pass the combustion products out through a nozzle,” Hallion says.
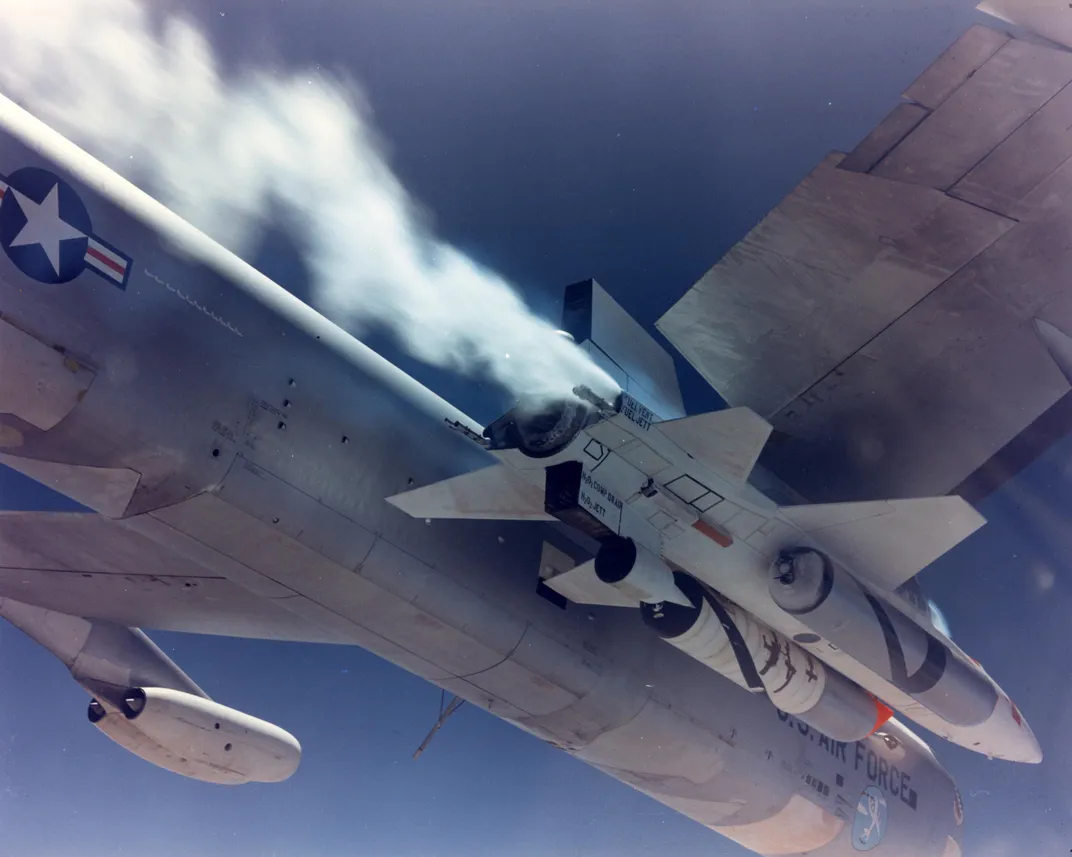
“The only problem is that the classic ramjet has a flow of air through it that is subsonic,” he says. As a ramjet passes Mach 5, the shock waves at the engine inlet slow the air down and also heat it so much that efficient combustion breaks down. The solution to that limitation is to once again get out of the way of the air, according to Lewis, using a supersonic-combustion ramjet engine, or “scramjet.”
“In a scramjet, the air comes into the engine and you don’t compress it,” says Lewis. “You design the inlet so it keeps moving at supersonic speed.” The temperatures don’t rise as high as with a ramjet and “you can continue to inject fuel, mix it and burn it and get useful thrust.”
In 1958, when ramjets were being used in the United States as missile propulsion systems, Richard Weber and John MacKay published the first comparison of ramjet engines with the then-theoretical scramjet. The men were assigned to the Lewis Flight Propulsion Laboratory (then part of the National Advisory Committee for Aeronautics; today it is the NASA Glenn Research Center). NASA hoped to fly a research scramjet on the X-15A-2, but during a 1967 test flight, the skin of the X-15 became so hot that the attachment holding a test model of the scramjet to the underside of the X-15’s aft fuselage melted and the model dropped off the airplane. A complete version never flew.
It would not be until 2004 and the X-43 that a scramjet would officially fly. Some dispute the X-43’s primacy because of an earlier successful scramjet experiment. In 2001 and 2006, a scramjet program at the University of Queensland in Australia called HyShot successfully ignited a scramjet attached to a rocket, but it didn’t produce thrust that was greater than drag. Says Lewis: “It wasn’t designed to have thrust greater than drag. It was an engine experiment on a larger rocket and they specifically didn’t want a lot of thrust because it would have thrown the rocket off. What they were trying to show was that they could burn fuel in a supersonic stream inside a scramjet, and they did that.” But the X-43 went one better: It produced thrust greater than drag in 2004, reaching a speed on a third test flight of about 7,000 mph at 110,000 feet—almost Mach 10—for 11 seconds.
Theoretically, there’s no speed limit to a scramjet, Hallion says. “You could take that scramjet all the way up to orbital velocity [Mach 25], as long as you could provide fuel to it and some sort of air.”
And that’s just what was tried with the National Aero-Space Plane program, announced by President Ronald Reagan in his 1986 State of the Union address. What became known as the X-30 was designed to be a single-stage-to-orbit spaceplane powered by scramjet engines that would at high altitude function more like ram-rockets, liquefying the air and mixing it with fuel. “People found that you can get up to Mach 12, Mach 15,” Hallion says. “But you know, you find when you’re trying to get those last several thousand feet per second to get you to orbital velocity, it just becomes a tremendous time waster. And money waster.”
The National Aero-Space Plane was canceled in 1994, far over budget and with key technologies still immature. “Hypersonics became associated in people’s minds with very big, expensive, complex programs that broke your heart,” says Hallion.
In the early 2000s, Russian researchers claimed to have flown the first scramjet engine. “It looks amazingly like that engine we were planning to fly on the X-15 rocketplane that we never did,” says Lewis. “There’s a debate as to whether their engine worked or not. The data was inconclusive.”
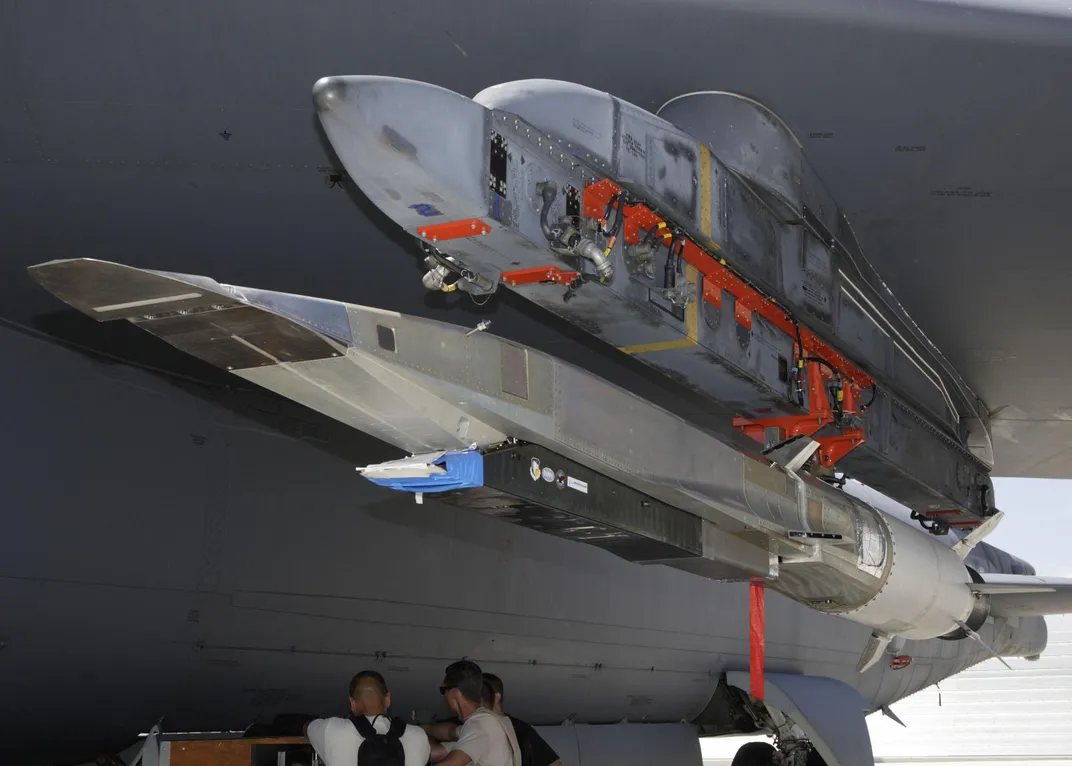
One of eight hypersonic wind tunnels in the Arnold Engineering Development Complex, in locations spread around the country, Tunnel 9 is also unique. Each facility specializes in one aspect of essential testing, all working in combination to provide data that can be used in computer models, Marren says. For instance, there are facilities in Tullahoma, Tennessee, where researchers can actually ignite a scramjet in a wind tunnel or test the thermal protection designs. “Here at Tunnel 9, our job is to get the highest Mach that we have in our facilities and also to get the most realistic pressures,” he says.
In the Tunnel 9 building lobby, there is a small museum. The original German-built Tunnel 1 wind tunnel test section is there, as are steel test models that have been used in Tunnel 9. There’s the simple cone of a ballistic missile warhead. There’s a model of the space shuttle. And then there’s a sharply angled delta shape with a slight dorsal ridge and a slight ventral groove. It’s almost an arrowhead shape, and like an arrowhead, it was made by knapping a larger object. “You start with a cone, and a cone has high drag,” Marren says. “If you take its flow field and you cut out the portions that you don’t need, computationally, you’re left with only the portions that can actually build high pressure underneath, which gives it lift.” In other words, take the cone, cut out anything that causes drag but does not contribute lift, and this is the shape you end up with. The result is a waverider, a geometry that can ride its own shock wave, and a key technology in contemporary hypersonics.
The X-43 was a direct offshoot of the canceled X-30, initially intended as a subscale aerodynamic and scramjet demonstrator. It reached Mach 9.6, but “the most exciting news about that flight is that the scramjet worked,” Lewis says. It worked just as it had been conceived it would in the 1950s. The only drawback? It wasn’t a “thermally balanced” engine, meaning the engine could run only a handful of seconds before the walls would have started melting. The limited experimental program was chalked up as a success.
Then, from 2010 to 2013, the Air Force conducted four test flights of the X-51, another uncrewed, scramjet-powered test vehicle, this time designed as a waverider. In the most successful of the four flights, the X-51 flew until it ran out of gas, 209 seconds after launch. It was the longest sustained scramjet flight ever conducted, and it was the “Lindbergh moment,” according to Lewis. “X-51 showed that it actually works.”
Referencing Lindbergh isn’t just being poetic. Hallion also believes that the X-51 has now done for air-breathing, hypersonic engines what Lindbergh’s 1927 crossing of the Atlantic did for the radial piston engine. “That was a tremendous indication of reliability,” Hallion says. “I would say we’re beyond the very promising stage, to the point that now we’re actually looking at implementation.”
That will likely mean missile technologies at first, along with other military applications, Hallion says. But are we anywhere closer to a hypersonic airliner? “Yes,” he says. “The answer is clearly yes.”
/https://tf-cmsv2-smithsonianmag-media.s3.amazonaws.com/filer/bc/1d/bc1d86cd-91fb-4946-a515-29233266781a/05u_dj2020_hawcconcept_live.jpg)
Bowcutt went to work for Rockwell International (which was then purchased by Boeing) conducting engine-airframe integration for the X-30 National Aero-Space Plane. He also worked on scramjet integration and design optimization until the spaceplane was canceled. He learned lessons along the way that helped him contribute to both the X-43 and X-51 programs. Only a few of his colleagues remained in hypersonics research, but some, Bowcutt notes, still work in hypersonics at Boeing and elsewhere. Computer design and simulation tools have come a long way since the X-30, as have materials science and onboard electronics.
Now Bowcutt is back where he started, developing plans for a hypersonic airliner, a Mach 5 cruiser capable of crossing the Pacific in three hours. “If you dial back to Mach 5—a lot of the technologies now exist to put together a Mach 5 airplane,” he says. The proposed airplane wouldn’t use scramjets, which are still considered an immature technology. “The direction we’re going is a turbo-ramjet, where it’s an integration of some kind of turbofan engine with a ramjet and it transitions from one to the other.”
Hypersonic aircraft designs have to be highly integrated, Bowcutt says. The engines and the wings and the fuselage must all blend together—unlike the tube-and-wing airliners of today. “It’s not like, here’s a wing and here’s a body and here’s some engines and you bolt it all together,” he says. Although he cannot reveal Boeing’s design yet, the vehicle’s engines and structure must be highly integrated, and the result may resemble a closely integrated waverider. “What I envision is this highly blended waverider-type vehicle,” Bowcutt says. “They are aerodynamically efficient at hypersonic speed.”
It’s a very real proposal, Bowcutt says, although he admits it’s not right around the corner. “Technologically, this is something we can do,” he says. “But there’s regulatory, there’s business case. When all those things come together, we envision that this is 20-plus years away.”
Of course, a 20-year development timeline still depends on the continued pursuit of air-breathing hypersonic technology. NASA did not follow up on the successful X-43 and X-51 programs, and the Pentagon has largely gone in a different direction. Most of the hypersonic weapons systems now being developed in the United States are not scramjet-powered but “boost-glide” systems, Bowcutt says. The designers use a rocket to boost a maneuverable wave-riding vehicle to high altitude; it then glides at Mach 20 to a target. In 2011, the DARPA Hypersonic Technology Vehicle 2 (HTV-2) managed controlled flight at Mach 20 for several minutes before crashing into the Pacific. The intense heat at that speed peeled its skin off, preventing stable flight.
On the air-breathing side, the DARPA/Air Force Hypersonic Air-breathing Weapon Concept, or HAWC, is building on X-51 technology with the aim of producing a hypersonic cruise missile. In terms of air-breathers, says Mark Lewis, “that’s kind of it.”
Rocket-accelerated boost-glide weapons are the obvious choice for long-range targets, Lewis says. Air-breathing weapons have advantages at shorter ranges: They can be packaged more compactly since they don’t need to carry oxidizers with them, can fly at lower altitudes, and have already been test-flown at a tactical scale in the X-51.
DARPA director Steven Walker said last May that the Pentagon is “aggressively pursuing” hypersonic technology. Both HAWC and a weapon dubbed Tactical Boost-Glider, a follow-on to the HTV-2, are due to fly in coming months. A small new air-launched rocket, Generation Orbit’s X-60, passed its Critical Design Review in March; it is intended to fly hypersonic experiments much more frequently than before. “I’m fond of saying that we entered the 19th century at six miles an hour; that was the speed of an animal-drawn vehicle,” Hallion says. He notes that we entered the 20th century at the 60 mph of a steam locomotive, the 21st at the 600 mph of an airliner. “We are absolutely on track, maybe even a little bit ahead of schedule, to enter the next century at 6,000 miles an hour,” he says.
But there is no guarantee that the United States will lead that progression. Russia has made hypersonics a military priority, and India is developing hypersonic cruise missile technology. Lewis notes that China is building wind tunnels and its researchers are publishing a mass of papers on hypersonics.
Back at Tunnel 9, Marren doesn’t appear worried that U.S. hypersonics research is going to fall off anytime soon. “We doubled our workload last year and need to double it again,” he tells me, while showing off images taken of a model Orion space capsule coated with a special thermal-sensitive paint that changes color with heat. It’s a recent innovation. Blue Origin and Boeing are both recent visitors, Marren says, and the Department of Defense is a frequent customer, though he declines to elaborate with program names. He first came to work here in 1984 and doesn’t plan to leave. “I love it,” he says.
Tunnel 9’s Super Lungs
To achieve Mach 18 at the right pressure, aerodynamicists can’t just stick a fan at one end of a tunnel. Instead, Tunnel 9 director Dan Marren says, his facility uses a more advanced version of the engineering pioneered at Nazi rocket facility Peenemünde, the “blow down” wind tunnel. Marren likens the concept to a balloon. “As soon as you open up the balloon, the air wants to rush from high pressure inside the balloon to the room,” he says. “So that air rushes out of the balloon as soon as you open it up. That’s all we do.”
/https://tf-cmsv2-smithsonianmag-media.s3.amazonaws.com/filer/a8/0d/a80d3c5a-87bf-454e-9e26-f458b267578d/05o_dj2020_whiteoaktunnel_live.jpg)
The vacuum sphere sits at the end of the blow down wind tunnel test circuit. At the beginning sits a pressure vessel that can compress nitrogen gas to 1,000 atmospheres. “Now I’ve got a thousand times more pressure times a thousand,” Marren says. “We’ve got a factor of a million.” Pressurized nitrogen rushes from the pressure vessel, through the test chamber, and into the vacuum sphere.
When the pressurized nitrogen is released, it is compressed through a small aperture, then allowed to expand in a nozzle that connects to the test chamber, Marren explains. The ratio between the size of the aperture and the nozzle determines the velocity of the airflow. “For, say Mach 14, it’s one-inch to five-foot diameter,” he says. “That will give you Mach 14. Half an inch to five-foot diameter gives you Mach 18.”
The 40-foot-long blue nozzle stretches from one end of a large, gymnasium-like chamber to the other. A small-scale model sits in the five-foot-diameter test section. All the humans are safely ensconced in an adjacent control room behind thick concrete walls. The tunnel can sustain Mach 18 airflows for up to 15 seconds and pitch a 200-pound model at 80 degrees a second, Marren says, collecting all the data they need. Test runs like this, along with other mathematical and computer tools, have kept hypersonics alive. —Jon Kelvey